The size-reduced Eudragit® RS microparticles prepared by solvent evaporation method – monitoring the effect of selected variables on tested parameters
Velikostně redukované mikročástice na bázi Eudragitu® RS připravené metodou odpaření rozpouštědla – sledování vlivu vybraných proměnných na testované parametry
Mikročástice se sníženou velikostí částic byly úspěšně připraveny metodou odpařování rozpouštědla. Pro přípravu každého vzorku byly použity různé parametry a byl hodnocen jejich vliv na výsledné mikročástice. Jako modelové léčivo byl pro enkapsulaci do částic na bázi Eudragit® RS vybrán nerozpustný ibuprofen. Získané mikročástice byly zhodnoceny pomocí optické mikroskopie a skenovací elektronové mikroskopie. Byl sledován vliv objemu vodné fáze (600, 400, 200 ml) a koncentrace polyvinyl alkoholu (1% a 0,1%) na vlastnosti mikročástic, jako je enkapsulační účinnost, drug loading, burst effect a morfologie mikročástic. Bylo zjištěno, že vzorek připravený s 600 ml vodné fáze a 1% koncentrací PVA poskytoval nejpříznivější výsledky.
Klíčová slova:
mikročástice • odpaření rozpouštědla • prodloužené uvolňování léčiva • Eudragit RS®
Authors:
Kalliopi Vasileiou; Jakub Vysloužil; Miroslava Pavelková; Jan Vysloužil; Kateřina Kubová
Published in the journal:
Čes. slov. Farm., 2017; 66, 274-280
Category:
Původní práce
Summary
Size-reduced microparticles were successfully obtained by solvent evaporation method. Different parameters were applied in each sample and their influence on microparticles was evaluated. As a model drug the insoluble ibuprofen was selected for the encapsulation process with Eudragit® RS. The obtained microparticles were inspected by optical microscopy and scanning electron microscopy. The effect of aqueous phase volume (600, 400, 200 ml) and the concentration of polyvinyl alcohol (PVA; 1.0% and 0.1%) were studied. It was evaluated how those variations and also size can affect microparticle characteristics such as encapsulation efficiency, drug loading, burst effect and microparticle morphology. It was observed that the sample prepared with 600 ml aqueous phase and 1% concentration of polyvinyl alcohol gave the most favorable results.
Key words:
microparticles • solvent evaporation • sustained drug release • Eudragit RS®
Introduction
Control drug delivery systems continue to have undoubtedly high impact in the treatment of diseases. Since the beginning of the nineties, several approaches and studies have been made to achieve more sophisticated drug delivery systems. A very important role of a drug delivery system is the successful delivery of medications into specifically targeted parts, such as small tissues, in sufficient amount and at the appropriate rate1). To achieve this goal, scientists have turned to both nano and microparticle technology2).
Microparticles can be characterized as a well-studied drug carrier with great pharmacokinetic features in comparison with other drug carriers3). Also, it has been presented in various applications ranging from biotechnology to construction materials having an outstanding potential in many different fields4). A number of techniques have been reported for microparticle preparation including solvent evaporation method. According to drug characteristics, preparation is mostly performed either by single (w/o)5) or double emulsion (w/o/w) method modification6). For hydrophobic drugs single emulsion process is used5) while for hydrophilic drugs the double emulsion method is preferred 6). In the single emulsion method, the oil phase consists of a drug and a polymer which are dissolved in an organic solvent. The resulting emulsion is then dispersed in an aqueous phase containing a dissolved emulsifier. The main role of the emulsifier is to promote stability in the dispersed phase and inhibit coalescence and flocculation of microparticles7). In the end of the evaporation, microparticles are isolated and dried8). Different polymers such as polylactic acid9), poly(lactic-co-glycolic acid)10) and polycaprolactone8) have been used for the encapsulation of various active drugs in solvent evaporation method.
A variety of techniques have been used in order to accomplish dispersion of the oil phase to the continuous phase. A common method is using a propeller acting as a stirrer11). With higher stirring speed, the size of the drops decreases. Homogenization is also being used for the production of an emulsion. The mixture is stirred by a homogenizer in high speed until the formation of a fine emulsion, resulting in much smaller size than produced by conventional agitation. There are many other methods being used for decreasing the size of microparticles such as sonication12), potentiometric dispersion and a use of a microfluidizer for the production of micro – emulsions13).
The main objective of the following experiment was the successful preparation of size-reduced microparticles using solvent evaporation method by applying a pre-emulsion step. Different parameters and their influence such as volume of aqueous phase and concentration of the emulsifier were observed.
Experimental section
Materials
Ibuprofen (Zentiva, k.s., Prague, Czech Republic) served as a model drug, Eudragit® RS was used for the formation of polymer matrix in oil phase. Dichloromethane – DM (Penta, Prague, Czech Republic) was the organic solvent used for the oil phase and polyvinyl alcohol – PVA (Mw 31.000–50.000; 98–99% hydrolyzed) (Sigma Aldrich, St. Louis, USA) served as an emulsifier. All materials were of Ph. Eur. quality.
Microparticle preparation
Microparticles containing ibuprofen were obtained by single emulsion (o/w) solvent evaporation method. For the formation of the oil phase, 200 mg of ibuprofen and 800 mg of Eudragit® RS were weighted and dissolved in 5 ml of dichloromethane. The mixture was stirred by homogenizer Ultra-Turrax (T25 basic, IKA-Werke, Staufen, Germany) at 11 000 rpm for thirty seconds to ensure a formation of a fine micro emulsion (pre-emulsion step).
The resulted emulsion was then, all at once, emulsified into the aqueous continues phase, which was prepared from respective amount of PVA and purified water. Evaporation occurred under a mechanical stirrer (Heidolph RZR 2021, Sigma Aldrich, St. Louis, USA) at 600 rpm for 90 minutes. After evaporation the prepared microparticles were centrifuged (EBA 20 Hettich, Germany) at 50 rpm for 5 minutes and then collected through a vacuum filtration (Pragopor membrane filters – 0.23 μm) and dried at 25 °C in a cabinet drier (HORO – 048B, Dr. Hofmann GmbH, Ostfildern, Germany).
To compare the results with our previous method we prepared reference sample, in which the preparation process was almost identical apart from except the fact the pre-emulsion step was not employed and the particles were collected on fine 80 μm mesh sieve.
The prepared samples were named in accordance with the concentration of PVA and the volume of aqueous phase (See Table 1).
Microparticle characteristics
Drug content analysis
The ibuprofen content was determined by measuring the absorbance at 264 nm by an UV/Vis spectrometer (Lambda 25, Perkin Elmer, USA). The samples were prepared by dissolving an appropriate amount of dried microparticles in 25 ml of dichloromethane. This procedure was carried out in triplicate and the obtained results were expressed as mean values and their standard deviations. From the obtained values encapsulation efficiency (%EE), drug load (%DL) and practical yield were determined by using the equations below14–16):
Encapsulation efficiency:
Drug loading:
Yield:
Where w1 represents the actual weight of drug in microparticles, ct is the theoretical amount of drug, w2 is the total weight of microparticles and wt is the theoretical yield (total amount of drug and polymer used for the microparticle preparation). EE and DL procedures were carried out in triplicate.
Optical microscope analysis
200 microparticles of the reference sample (R) were analyzed by NIKON SMZ 1500 stereo microscope (Nikon, Tokyo, Japan) and 72AUC02 USB camera (The Imaging Source, Bremen, Germany). 200 microparticles of each of the remaining samples were analyzed by NIKON ECLIPSE E200 (Nikon, Tokyo, Japan) and the results were evaluated by computer software NIS-Elements AR 4.0 (Nikon, Tokyo, Japan). From the measurement sphericity factor and equivalent diameter data were obtained. A picture of every sample was taken.
Scanning electron microscopy
The morphology of microparticles was evaluated by a scanning electron microscopy. The samples were coated fifteen times for the avoidance of charging artifacts. The coating procedure took part with a 10 nm thick layer of platinum/palladium (Pt/Pd) by the ion sputtering coating method with argon atmosphere. Pt/Pd was sputtered at a current of 20 mA for 20 seconds (Cressington sputter coater 208HR, England). The samples then were placed in a SEM sample holder using carbon conductive double-sided adhesive discs (EMS, USA). Pictures were taken by a Hitachi SU8010 (Hitachi High-Technologies, Japan) scanning electron microscope at an accelerating voltage of 14.0 kV for 40 seconds.
In vitro release studies
Ibuprofen loaded microparticles were tested regarding their in vitro drug release kinetic profiles in an automated dissolution device (SOTAX AT 7 On-Line System, Donau Lab, Zurich, Switzerland) at 75 rpm. The temperature was kept at 36.0 ± 0.5 °C. The dissolution medium that was used in the test was 500 ml of 6.8 pH buffer. Sustained drug release rate was expected since ibuprofen is practically insoluble in water. A sufficient quantity of microspheres containing ibuprofen was weighted with respect to the actual drug content and was placed in the apparatus. Analysis of the samples occurred by an UV spectrophotometer (Lambda 25, Perkin Elmer, St. Louis, MO) at 222 nm. The given data were correlated in accordance with the equations of the mathematical models of drug release studies17):
Zero order equation:
First order equation:
Higuchi model:
Korsmeyer-Peppas equation:
Hixson-Crowell model:
Baker-Lonsdale model:
where Mt is the amount of drug released in time t; M∞ is the absolute cumulative amount of drug released at an infinite time; K0, K1, KH, KKP, KS and KBL are the zero order, first order, Higuchi, Korsmeyer-Peppas, Hixson Crowell and Baker-Lonsdale release constants. Release exponent n of the Korsmeyer-Peppas model characterizes the mechanism of the drug release: n = 0.5 corresponds to the Fickian diffusion, 0.5 < n < 1.0 to an anomalous transport, n = 1.0 to the zero-order release kinetics and n > 1.0 to the super Case II transport.
The similarity factor f2 was designed in order to compare dissolution profiles between original and generics18, 19). Therefore, it can also be applied as an equation to determine a possibility of dependence. The range of the similarity factor f2 can range between 0 and 100. If the value is 50 or higher than 50 (f2 > 50), then can be said that the drug release profiles are more than 90% similar. While if this value is lower than 50 (f2 < 50) then the drug release profiles are not similar and the observed influence of the process variables is considered as significant.
Similarity factor:
where Ti is the drug amount (%) released at time interval i in the tested sample, Ri is the drug amount (%) released at time interval i in the reference sample, n is the total number of samplings.
Results and discussion
Encapsulation process
Drug content analysis results are displayed in Table 2. Encapsulation efficiency was in the range of 35.85 to 49.16% for the tested samples, for the reference sample was 31.32%. The change of the aqueous phase volume had significant influence on the %EE. While it was reported before, that a smaller volume of aqueous phase is more favorable to achieve higher drug encapsulation20), in this experiment the samples prepared with 600 ml of PVA solution exhibited higher EE than the samples with lower volume of aqueous phase, partly because of higher polymer adherence to stirrer (and therefore higher losses) in smaller volume samples. It was also observed that increased concentration of PVA lead to lower encapsulation yields which can be related with a higher viscosity presented in aqueous phase21, 22).
The range of drug loading values was from 14.81% to 26.56%. The indication in previous studies, that smaller microspheres demonstrated higher values because they tend to have larger surface area for encapsulation, was also observed in our study23, 24). Free drug crystal formation in the aqueous medium and adherence of the polymer to the stirrer can explain the drug loss which was even more visible in samples with lower aquatic phase volume. This can also explain the lower yield in those samples25). Generally samples with low concentration of PVA had higher %DL values, which is in agreement with the literature26).
The highest yield results were demonstrated by the sample which contained concentration of 1% PVA and 600 ml aqueous phase. The sample that contained 0.1% PVA and 600 ml aqueous phase showed also a quite high value with yield 53.7%. The rest samples ranged between 31.6 to 47.6%. Low yield values can be also explained due to a partial adherence of the polymer on the stirrer and more importantly adherence on beaker walls25).
The best overall results were demonstrated by the sample which contained concentration of 1% PVA and 600 ml aqueous phase.
Optical microscope analysis
In table 2 sphericity factor and equivalent diameter are also presented. Images of prepared and finished microparticles are shown in figure 1. Generally, all the samples demonstrated great sphericity, with the sphericity factor ranging from 0.971 ± 0.026 to 0.986 ± 0.020, results that were similar to the R sample. Microparticles prepared pre-step had significantly lower size than the reference, ranging between 2.93 ± 0.99 to 6.96 ± 2.5 while the reference sample was 202.17 ± 35.06 μm. It clearly demonstrates significant size decrease because of the pre-emulsion step. It has been reported that the size of microparticles can be affected by the volume of aqueous phase, the temperature, viscosity, stirring speed and the amount of the used emulsifier27, 28). PVA, as a high molecular weight polymer, could affect the dispersion phase by increasing the viscosity. Therefore, the reduction of the emulsion particles to smaller size is more difficult29, 30). In this study, size distribution of the samples did not show major deviations. In samples prepared with 0.1% PVA the particle size was slightly decreasing with lower water phase volume, in samples prepared with 1% PVA we do not observe this trend.
Scanning electron microscopy
The microparticles were spherical, moderately porous with linear outer surfaces. The absence of higher porosity in the surface of the microspheres can be naturally explained by the fact that a porogenous substance was not employed during the preparation of the samples. The slight formation of several pores per particle can be furthermore explained because of the diffusion of the inner phase, which can contribute to the development of the pores31). It was also noticed that concentration of PVA slightly affected the porosity. As it can be seen in Figure 2, lower PVA concentration led to higher particle porosity which is also in agreement with literature32). The physical morphologies of the microparticles prepared with higher PVA concentration were similar. On the other hand at lower PVA concentration polymeric particles from Eudragit® RS had much higher tendency to stuck together into larger polymeric blocks, as can be seen in sample E0.1-600 (See Figure 2 e, f).
Drug release behavior
The drug dissolution profiles were tested for samples R, E1-600, E1-200 and E0.1-600 and they are shown in Figure 3. The test was performed only in the mentioned samples due to shortage of material after the previous tests. The dissolution analysis lasted 720 minutes for each sample. All the samples except sample R were characterized by a detectable burst effect, which was followed by a sustained drug release rate that lasted until the end of the dissolution test. This probably happened due to the release of surface adhered drug33). Generally, the burst effect is considered undesirable, because it can lead to a local or systemic toxic effect34, 35). On the contrary, in some cases such as in wound treatment, it is preferred to induce quickly therapeutic dose level36).
Sample E1-600 is an anomaly among the other samples, as it quickly released 40.75% of the drug during the first 10 minutes and by the end of the test drug release reached 68.61%. However there is a chance that the striking burst effect was a consequence of coincidental drug cumulation on particle surface. The other samples showed notably slower release. Reference R sample did not produce burst effect and had released 19.97% of drug content by the end of 720 minutes. 30.27% of the drug was released from the E1-200 sample by the 720 minutes mark. Finally the sample E0.1-600 showed a 38.50% release after 720 minutes. This observation can be elucidated because Eudragit® RS used for the microsphere preparation is insoluble semipermeable polymer, which makes it more difficult for the dissolution medium to dissolve the drug in the miscrospheres33). In addition, the R sample, as being bigger sized, had lower surface to weight ratio, leading to lower diffusion rate due to smaller contact area with the dissolution buffer33). Porosity has also a significant effect on the drug release behaviour37). Pores on the microparticle surface may greatly increase the drug release rate37). This could also partially explain the higher release rate in the samples prepared with 600 ml water phase, due to higher porosity on their surface in comparison with the sample prepared in 200 ml of water phase, as being observed in Figure 2.
The drug release from microspheres can occur by various mechanisms including physical erosion, hydrolysis of the polymer, ion – exchange mechanism, diffusion through the surface pores or through the polymer, or by a combination of those mechanisms38, 39). The obtained release data were examined by using the kinetics equation for the determination of release kinetics and mechanisms. Table 3 presents the determination coefficient values R2 for the applied models. The obtained data poorly corresponded with the first order kinetic model, while a good fitting was found with Baker-Lonsdale model with the results of R2 ranging from 0.94 to 0.99, and also with Hixson-Crowell model with values between 0.93 and 0.99. It was observed that microspheres release profiles showed a biphasic composition. First phase was an initial release due to the drug released from the particle surface, the second was a constant sustained drug release. This scenario characterizes the drug release by surface erosion which can be related with the lack of porosity in the microspheres surface40). This can be also confirmed visually from Figure 2. Interesting results came from Higuchi model. The good association with Higuchi model (except R sample) can confirm Fickian diffusion as a part of the drug release mechanism from ibuprofen particles. The behavior of the examined samples suggests probable partial participation of Fickian diffusion as well as swelling behavior because Eudragit® RS tends to be generally insoluble41).
Similarity factor analysis was also calculated for the tested samples which are presented in Table 4. As it is observed the majority of the compared samples showed no similarity with values lower than 50. Similarity was confirmed only between sample E0.1-600 – E1-200 with 66.75 and E1-200 – R with 54.55, respectively. The low f2 values suggest that the observed parameters can have significant effect on the drug release.
Conclusion
Size-reduced ibuprofen loaded microparticles were successfully prepared by o/w solvent evaporation technique. In the present study for the formation of microparticles we applied different parameters and evaluated all the samples. All of the prepared microparticle samples gave very high values of sphericity factor. Samples prepared with 1% PVA concentration had higher yields, which confirms, that the PVA concentration may co-influence produced yields. In terms of drug loading low PVA concentration samples were the ones having higher values, which is in accordance with the literature. As it was found the PVA concentration also influenced the drug release profiles when the dissolution test occurred, a finding that was expected. The dissolution test took part by applying various kinetic models and the results demonstrated that ibuprofen was probably released by a partial participation of Fickian diffusion. In overall, the sample which had the most favorable traits was E1-600. It was concluded that the applied parameters influenced the microparticle characteristics. The results show the complexity of the process working with solvent evaporation method.
Conflicts of interest: none.
Received August 23, 2017
Accepted November 11, 2017
K. Vasileiou
PharmDr. Jakub Vysloužil
M. Pavelková
K. Kubová
Department of Pharmaceutics, Faculty of Pharmacy
University of Veterinary and Pharmaceutical Sciences
Palackého 1/3, 612 42 Brno, Czech Republic
e-mail: vyslouzilj@vfu.cz
Jan Vysloužil
Department of Biochemistry, Faculty of Science, Masaryk University
Zdroje
1. Panicker Varuna P., Anu Gopalakrishnan. Advances in drug delivery systems 2014.
2. Schmidt C., et al. Nano-and microscaled particles for drug targeting to inflamed intestinal mucosa – A first in vivo study in human patients. Journal of controlled release 2013; 165(2), 139–145.
3. Chowdhury S., et al. An overview of drug delivery vehicles for cancer treatment: Nanocarriers and nanoparticles including photovoltaic nanoparticles. Journal of Photochemistry and Photobiology B: Biology 2016; 164, 151–159.
4. Campos E., et al. Designing polymeric microparticles for biomedical and industrial applications. European Polymer Journal 2013; 49(8), 2005–2021.
5. Beck L. R., Cowsar D. R., Lewis D. H., Gibson J. W., Flowers C. E. New long-acting injectable microcapsule contraceptive system. Am J Obstet Gynecol. 1979; 135, 419–426.
6. Iqbal M., Zafar N., Fessi H., Elaissari A. Double emulsion solvent evaporation techniques used for drug encapsulation. International journal of pharmaceutics 2015; 496(2), 173–190.
7. Padalkar A. N., Sadhana R. S., Thube M. W. Microparticles: an approach for betterment of drug delivery system. Int. J. Pharm. Res. Dev. 2011; 1, 99–115.
8. Iqbal M., et al. Double emulsion solvent evaporation techniques used for drug encapsulation. International journal of pharmaceutics 2015; 496(2), 173–190.
9. Bodmeier R., McGinity J. W. Solvent selection in the preparation of poly (DL-lactide) microspheres prepared by the solvent evaporation method. International journal of pharmaceutics 1988; 43(1–2), 179–186.
10. Patel R. S., et al. Doxycycline delivery from PLGA microspheres prepared by a modified solvent removal method. Journal of microencapsulation 2012; 29(4), 344–352.
11. Han N. S., Basri M., Abd R. M., Abd R. R., Salleh A. B., Ismail Z. Preparation of emulsions by rotor-stator homogenizer and ultrasonic cavitation for the cosmeceutical industry. Journal of cosmetic science 2012; 63(5), 333–344.
12. Kim K. K., Pack D. W. Microspheres for drug delivery. BioMEMS and Biomedical Nanotechnology. Springer US 2006; 19–50.
13. O’Donnell P. B., McGinity J. W. Preparation of microspheres by the solvent evaporation technique. Advanced drug delivery reviews 1997; 28(1), 25–42.
14. Gentile P., et al. Localised controlled release of simvastatin from porous chitosan–gelatin scaffolds engrafted with simvastatin loaded PLGA-microparticles for bone tissue engineering application. Materials Science and Engineering: C 59 2016; 249–257.
15. Liu X., Sun Q., Wang H., et al. Microspheres of corn protein, zein, for an ivermectin drug delivery system. Biomaterials 2005; 26, 109–115.
16. Saravanan M., Bhaskar K., Maharajan G., Pillai K. S. Ultrasonicallycontrolled release and targeted delivery of diclofenac sodium via gelatin magnetic microspheres. Int. J. Pharm. 2004; 283, 71–82.
17. Shaikh H. K., Kshirsagar R. V., Patil S. G. Mathematical models for drug release characterization: a review. World J. Pharm. Pharmacent. Sci. 2015; 4, 324–338.
18. Berkland C., Kim K. K., Pack D. W. PLG microsphere size controls drug release rate through several competing factors. Pharmaceutical research 2003; 20(7), 1055–1062.
19. Esbensen K. H., Guyot D., Westad F., Houmoller L. P. Multivariate data analysis: in practice: an introduction to multivariate data analysisand experimental design. Oslo: CAMO AS Publications 2004.
20. Jelvehgari M., et al. Control of encapsulation efficiency in polymeric microparticle system of tolmetin. Pharmaceutical development and technology 2010; 15(1), 71–79.
21. Capan Y., Woo B. H., Gebrekidan S., Ahmed S., DeLuca P. P. Influence of formulation parameterson the characteristics of poly(D, L-lactide-co-glycolide) microspheres containing poly(L-lysine)complexed plasmid DNA, J. Control. Release 1999; 60, 279–286. doi: 10.1016/S0168-3659(99)00076-0
22. Mao S., Shi Y., Li L., Xu J., Schaper A., Kissel T. Effects of process and formulation parameterson characteristics and internal morphology of poly(d,l-lactide-co-glycolide) microspheres formedby the solvent evaporation method. Eur. J. Pharm. Biopharm. 2008; 68, 214–223. doi: 10.1016/j.ejpb.2007.06.008
23. Chen W., Palazzo A., Hennink W. E., Kok R. J. The effect of particle size on drug loading and release kinetics of gefitinib-loaded PLGA microspheres. Molecular Pharmaceutics 2016.
24. Čalija B. (ed.) Microsized and nanosized carriers for nonsteroidal anti-inflammatory drugs: formulation challenges and potential benefits. Academic Press 2017.
25. Thies C. Microcapsules and nanoparticles in medicine and pharmacy. Boca Raton: CRC press 1992.
26. O’Donnell P. B., McGinity J. W. Preparation of microspheres by the solvent evaporation technique. Adv. Drug Deliv. Rev. 1997; 28, 25–42. doi: 10.1016/S0169-409X(97)00049-5
27. Freiberg S., Zhu X. X. Polymer microspheres for controlled drug release. International journal of pharmaceutics 2004; 282(1), 1–18.
28. Yan Ch., et al. Characterization and morphological analysis of protein-loaded poly (lactide-co-glycolide) microparticles prepared by water-in-oil-in-water emulsion technique. Journal of Controlled Release 1994; 32(3), 231–241.
29. Khan A. U., Nasir M. A., Nasir M. Rheological studies on stabilised zirconia aqueous suspensions. Journal of Colloid Science and Biotechnology 2012; 1(2), 175–184.
30. Ibraheem D., et al. Effects of process parameters on the colloidal properties of polycaprolactone microparticles prepared by double emulsion like process. Colloids and Surfaces A: Physicochemical and Engineering Aspects 2014; 445, 79–91.
31. Cai Y., Chen Y., Hong X., Liu Z., Yuan W. Porous microsphere and its applications. International journal of nanomedicine 2013; 8: 1111.
32. Mao S., et al. Effect of WOW process parameters on morphology and burst release of FITC-dextran loaded PLGA microspheres. International journal of pharmaceutics 2007; 334(1), 137–148.
33. Nath, Bipul, Lila Kanta Nath, Pradeep Kumar. Preparation and in vitro dissolution profile of zidovudine loaded microspheres made of Eudragit RS 100, RL 100 and their combinations. Acta Pol Pharm 2011; 68(3), 409–415.
34. Yeo Y, Park K. Control of encapsulation efficiency and initial burst in polymeric microparticle systems. Archives of pharmacal research 2004; 27(1), 1.
35. Huang X., Brazel C. S. On the importance and mechanisms of burst release in matrix-controlled drug delivery systems, J. Control. Release 2001; 73, 121–136.
36. Huang X., Brazel C. S. On the importance and mechanisms of burst release in matrix-controlled drug delivery systems. Journal of controlled release 2001; 73(2), 121–136.
37. Yang Yi-Yan, et al. Effect of preparation conditions on morphology and release profiles of biodegradable polymeric microspheres containing protein fabricated by double-emulsion method. Chemical Engineering Science 2000; 55(12), 2223–2236.
38. Ramirez L. Biodegradable poly (DL-lactic-co-glycolic acid) microspheres containing tetracaine hydrochloride. In-vitro release profile. Journal of microencapsulation 1999; 16(1), 105–115.
39. Herrero-Vanrell R., Refojo M. F. Biodegradable microspheres for vitreoretinal drug delivery. Advanced drug delivery reviews 2001; 52(1): 5–16.
40. Yin C., Li X. Anomalous diffusion of drug release from a slab matrix: Fractional diffusion models. International journal of pharmaceutics 2011; 418(1), 78–87.
41. Akhgari A., Tavakol A. Prediction of optimum combination of Eudragit RS/Eudragit RL/ethyl cellulose polymeric free films based on experimental design for using as a coating system for sustained release theophylline pellets. Advanced pharmaceutical bulletin 2016; 6(2), 219.
Štítky
Farmácia FarmakológiaČlánok vyšiel v časopise
Česká a slovenská farmacie
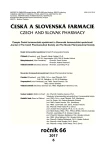
2017 Číslo 6
Najčítanejšie v tomto čísle
- Črevná mikrobiota: jej vývin a vzťah k vzniku niektorých ochorení
- Vývoj disolučnej metódy pre tablety s obsahom warfarínu sodného
- Karboxylové kyseliny z hnědých řas Fucus vesiculosus a Padina pavonica
- Velikostně redukované mikročástice na bázi Eudragitu® RS připravené metodou odpaření rozpouštědla – sledování vlivu vybraných proměnných na testované parametry