CROSSOVER FROM AUTOMATED TO MANUAL TITRATION OF FiO2 IN THE NICU: IS THERE A TRANSITION LAG?
Effective management of oxygenation of preterm infants in critical care profoundly impacts their outcome. Nurses are challenged to titrate the inspired oxygen in response to constant cardiopulmonary instability. Closed loop control of inspired oxygen based on continuous monitoring of oxygen saturation is just becoming available. Evaluating the relative effectiveness of closed loop control systems is complicated by the wide variability in manual control by nurses. This analysis explored the possibility of a lag in effective control associated with the transition from closed loop to manual control using data from a clinical crossover trial. A short but marked lag phase was detected. It is, however, unlikely to have impact on clinical care or crossover studies. Its presence highlights the anticipative nature of closed loop control as contrasted to the observative nature of manual control.
Keywords:
Neonatal critical care, oxygen control, closed loop oxygen control
Authors:
Thomas Bachman; Karel Roubík
Authors place of work:
Czech Technical University in Prague, Czech Republic
Published in the journal:
Lékař a technika - Clinician and Technology No. 2, 2016, 46, 37-40
Category:
Původní práce
Summary
Effective management of oxygenation of preterm infants in critical care profoundly impacts their outcome. Nurses are challenged to titrate the inspired oxygen in response to constant cardiopulmonary instability. Closed loop control of inspired oxygen based on continuous monitoring of oxygen saturation is just becoming available. Evaluating the relative effectiveness of closed loop control systems is complicated by the wide variability in manual control by nurses. This analysis explored the possibility of a lag in effective control associated with the transition from closed loop to manual control using data from a clinical crossover trial. A short but marked lag phase was detected. It is, however, unlikely to have impact on clinical care or crossover studies. Its presence highlights the anticipative nature of closed loop control as contrasted to the observative nature of manual control.
Keywords:
Neonatal critical care, oxygen control, closed loop oxygen control
Introduction
After decades of promising research the closed loop control of inspired oxygen (FiO2) based on pulse oximetry (SpO2) is finally becoming available in the neonatal ICU. [1, 2, 3] Its routine clinical adoption will dramatically reduce the challenging and frustrating task nurses face in adjusting inspired oxygen in preterm infants during their weeks of respiratory support. [1, 2, 3] Numerous studies of preterm infants have shown the criticality of maintaining oxygen saturation in a “sweet spot” to balance the risk of bad outcomes associated with both hyperoxemia and hypoxemia. [4] Therefore better control of SpO2, that has become associated with closed loop control, further offers the promise of improved outcomes. [1, 3]
The uptake of oxygen from the alveoli to the pulmonary capillaries is quite fast. Further the time constant of washing in inspired gases throughout the lung is certainly less than a minute. [5] In the neonatal ICU numerous factors have impact. The equipment dead space and time constants associated with changes in SpO2 in response to an adjustment of FiO2 in the ventilator were recently characterized during respiratory support in the neonatal ICU. [6] Of course the lag phase, from adjustment to first response, varies primarily according to the ventilator, breathing circuit dead space and other ventilator system considerations. Further, as would be expected, there is also variation in the time constant and gain associated with a change in inspired and arterial oxygen, based on the respiratory mechanics and pathophysiology of the infant. Most automated FiO2-SpO2 control systems measure SpO2 and adjust FiO2 much faster than the infant-ventilation response. [3] The opposite is true during manual control. However, one small study of manual and automated control suggested that quicker response, whether associated with auto or dedicated manual control, resulted in more effective control. [7] Nevertheless quicker increases must also be associated with quicker decreases when faced with hyperoxemia.
Some automated systems have also been characterized as having an anticipative component in their control algorithms. [3] As an example, they might consider the rate of change of the SpO2 in making a decision about the next FiO2 adjustment. In contrast, routine manual control would be characterized as observative. That is, the nurse waits to observe the complete response to a change in FiO2 before considering the need for another change.
Physiological crossover studies should always consider the kinetics of the therapeutic intervention, and employ an adequate washout period between interventions. Nearly all of the evaluations of automated FiO2-SpO2 control utilized a physiological crossover design. However, because the SpO2 response to changes in inspired oxygen was known to be quite rapid compared to the duration of the intervention periods, washout periods in these studies have been considered unnecessary.
The aim of this study was to determine if there was a clinically relevant crossover effect when switching from automated to manual FiO2-SpO2 control. We speculated that the transition from automated to manual control, by virtue of its observative character, would result in a distinct transition.
Methods
Source data from a large crossover trial was used. [8] This trial enrolled 80 preterm infants at 9 centers. The order of intervention was randomized, with 40 receiving manual control after automated control. The database included the subjects’ demographics as well as SpO2 data sampled every 5 seconds for the two 24-hour intervention periods. Five of the 40 cases that transitioned from automated control to manual control were randomly selected. In addition, for the purpose of subjective comparison, 5 of the other 40 cases that transitioned from manual to automated control were also randomly selected. SpO2 data were extracted for the hour before and after the transitions between automated and manual control.
The system used in the van Kaam trial [8] was the AVEA-CLiO2 (CareFusion, Yorba Linda CA, USA). This system had been commercially available in Europe for a number of years. The control algorithm continuously monitors FiO2 and SpO2 and makes a decision every second about whether a change in FiO2 is needed. The decision is based on a set of rules that vary depending on whether the SpO2 is below, within or above the set SpO2 control range. The rules, while varying depending on that range, all consider 6 parameters. These are the rate of change of SpO2, the direction of change, the time outside the target range, the absolute deviation from the target range, the reliability of the pulse oximetry signal and the baseline FiO2. More details of the control algorithm and system performance are available. [3, 8]
Mean SpO2 was prospectively defined as the primary metric of control. The proportion of times with possible hypoxemia and possible hyperoxemia were selected as secondary endpoints, that might be associated with a shift in the mean. Possible hypoxemia and hyperoxemia were define as SpO2<86% and SpO2>96% when not inspiring room air, respectively. The presence of a relevant transition/washout was subjective, determined graphically. A 2-minute rolling mean of the SpO2 was used to filter out the normal rapid variation in SpO2 to facilitate identification of a transition effect. A two-minute averaging was selected as nearly all desaturation episodes are less than 1 minute. [9]
Differences in the mean SpO2 were evaluated using a pooled two-sample t test. Differences in hyperoxemia and hypoxemia were evaluated using a Z-test of proportions. A P value of <0.05 was considered statistically significant. Statistical analyses were conducted using XLStat (v16.6.03, Addinsoft USA).
Results
The 10 subjects selected were preterm infants studied in 4 neonatal ICUs. The interquartile range of their demographics were as follows: estimated gestational age (25‒26 weeks), age and weight when studied (16.5‒27.8 days) and (0.97‒1.22 kg). Most were receiving noninvasive respiratory support (6 continuous positive airway pressure, 2 intermittent positive pressure). During this two-hour analysis period the interquartile range of the SpO2 was 91‒96% in the automated to manual group and 90‒95% in the manual to automated group.
Figures 1ab present the rolling average of the SpO2 over the two-hour analysis periods. As would be expected even with the rolling average the SpO2 is not stable. The transition to manual from automated control is clear with a marked drop in SpO2 (Figure 1a). A transition period of 10 minutes was selected after creating the charts. The slope of the rolling average at the boundaries to the 10-minute transition period suggests a precipitous transition. In contrast (Figure 1b) no transition period is apparent when switching to automated from manual control.
The actual means for all the 5-second data points in two 10-minute transition periods are compared to the subsequent 50 minutes and shown in Table 1. As suggested in Figure 1a, during manual control the mean SpO2 in the transition is significantly lower (2.4% SpO2, P<0.001). In contrast, consistent with Figure 1b, there was no apparent difference in mean SpO2 transitioning to automated control. The variation of SpO2 (standard deviation) was similar among the periods.
The lower mean SpO2 during the manual transition also impacted the distribution at SpO2 extremes. The percent time with a SpO2 reflective of a risk of hypoxemia was higher during the transition (16.9% vs 7.2%, P<0.001) and correspondingly less with a risk of hyperoxemia (15.7% vs 25.9%, P<0.001). The percent time with SpO2 between these two extremes, normoxemia, was however unchanged (67.4% vs 66.9%, P=ns).
Discussion
The maintenance of SpO2 at the beginning of manual control of FiO2 was different than during ongoing control, as hypothesized. A similar transition was not seen when starting automated control. In the clinical environment drops in SpO2 are usually a result of disordered breathing. Thus maintaining desired SpO2 levels necessitates intermittent increases in FiO2. During the manual control transition period the SpO2 was lower, consistent with the observational nature of manual control.
We found the transition period was about 10 minutes. Fathabadi et al, reported on the neonatal SpO2 response to changes based on 580 isolated increases in FiO2 in 47 subjects. [6] They reported a typical delay of 22 seconds (IQR 8‒40) before SpO2 started to increase. They also found that the 95% response to an increase in FiO2 was 39 seconds (IQR 7‒105). While they reported considerable variation among episodes and subjects, this data suggests that two or three adjustments, each occurring after observing the response to the last adjustment, nominally a minute, would be much shorter than the 10 minutes that was identified as the transition period. We speculate therefore that during manual control the periods of observation from initiation and subsequent FiO2 adjustments are much longer than the physiological responses. This is consistent with the multitasking required of neonatal nurses.
While the SpO2 transition to manual adjustment was marked, the rolling average SpO2 stayed within the range associated with normoxemia (SpO2 86‒96%). The shift to lower levels resulted, nevertheless, in significant differences in SpO2 exposure. Specifically, there was an increased exposure to potential hypoxemia and decreased exposure to potential hyperoxemia. Differences of this magnitude are certainly clinically relevant, if they were to persist for a significant amount of time. [9, 10] It is unlikely, however, that infrequent transitions associated with shift or staffing changes would occur frequently enough to impact accumulative exposure. However some part of the differences reported with staffing levels might be associated with transitions associated with attending to particularly unstable infants. [11]
It appears that the transition period would not have a material effect on the results of crossover studies of FiO2-SpO2 control systems. As an example, even in a study with 4-hour intervention periods a 10-minute transition would represent only 4.2% of the intervention time. Studies more typically have utilized 12 or 24-hour intervention periods.
This study has some limitations. The analysis is based on the response of 5 subjects. While they were picked at random from a larger population, selection of different subjects might have resulted in different results. The analysis also used the pooled response of the subjects, a larger study evaluating paired within-subject differences would provide information about the variation among subjects. Nevertheless the differences we found were clinically relevant and highly statistically significant. This data came from a controlled trial in which the caregivers knew their manual control was being studied. In such circum-stances one would expect the control would better, and thus the effect less pronounced than during routine care. The actual transition effect associated with shift changes should be studied at centers with appropriate patient data monitoring systems.
Conclusion
There appears to be a lag phase with a drop in SpO2 at the beginning of manual titration of FiO2. This transition phase is longer than just the physiological response would predict. Its duration and magnitude are not sufficient to have any impact on patient risk or study design.
Acknowledgement
The work was not supported by a research grant or contract.
Thomas Bachman MSc
Faculty of Biomedical Engineering
Czech Technical University in Prague
Faculty of Biomedical Engineering
Department of Biomedical Technology
nám. Sítná 3105, CZ-272 01 Kladno
&
Economedtrx
Lake Arrowhead, CA USA 92352-1269
E-mail: TBachman@me.com
Phone: +1 909 337-0828
Zdroje
[1] Claure N., Bancalari E. Closed-loop control of inspired oxygen in premature infants. Semin Fetal Neonatal Med. 2015; 20(3), p. 545-50.
[2] Wilinska M., Skrzypek M., Bachman T., et al. Using the automated FiO2-SpO2 control in neonatal intensive care units in Poland: a preliminary report. Developmental Period Medicine, 2015; XIX, 3(I), p. 263-70.
[3] Fathabadi O.S., Gale T.J., Olivier J.C., Dargaville P.A. Automated control of inspired oxygen for preterm infants: What we have and what we need. Biomedical Signal Processing and Control. 2016; 28, p. 9–18.
[4] Sola A., Golombek S., Bueno M.T., et al. Safe oxygen saturation targeting and monitoring in preterm infants: can we avoid hypoxia and hyperoxia? Acta Paediatr. 2014; 103(10), p. 1009-18.
[5] Sivan Y., Deakers T.W., Newth C.J. An automated bedside method for measuring functional residual capacity by N2 washout in mechanically ventilated children. Pediatr Res. 1990; 28(5), p. 446-50.
[6] Fathabadi O.S., Gale T.J., Lim K., et al. Characterisation of the oxygenation response to inspired oxygen adjustments in Preterm Infants. Neonatology 2016; 109, p. 37–43.
[7] Wilinska M., Bachman T., Swietlinski J., Wasko A. Quicker response results in better SpO2 control– a comparison of 3 FiO2-titration strategies in ventilated preterm infants. AAEM 2015, 22, (4), p. 736–40.
[8] van Kaam A.H., Hummler H., Wilinska M., et al. Automated versus Manual Oxygen Control with Different Saturation Targets and Modes of Respiratory Support in Preterm Infants. J Pediartr 2015; 167(3), p. 545-50.
[9] Poets C.F., Roberts R.S., Schmidt B. et al. Association between intermittent hypoxemia or bradycardia and late death or disability in extremely preterm infants. JAMA. 2015; 314(6), p. 595-603.
[10] Kaufman D.A., Zanelli S.A., Gurka M.J., et al. Time outside targeted oxygen saturation range and retinopathy of prematurity. Early Human Development. 2014; 90(2), p. 35–40.
[11] Sink D.W., Hope S.A., Hagadorn J.I. Nurse: patient ratio and achievement of oxygen saturation goals in premature infants. Arch Dis Child Fetal Neonatal Ed 2011; 96(2), p. 93–8.
Štítky
BiomedicínaČlánok vyšiel v časopise
Lékař a technika
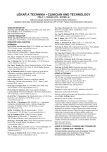
2016 Číslo 2
Najčítanejšie v tomto čísle
- CELL-BASED SENSOR CHIP FOR NEUROTOXICITY MEASUREMENTS IN DRINKING WATER
- EARLY DISCHARGE (48–72 HOURS) AFTER ACUTE ST-SEGMENT ELEVATION MYOCARDIAL INFARCTION: INTERIM RESULTS OF THE OPEN, RANDOMIZED, MONOCENTRIC STUDY
- TESTING A SYSTEM FOR PREDICTING MICROSLEEP
- DESIGN CONCEPTS FOR PREVENTING GAS BUBBLE INTERFERENCE IN MICROFLUIDIC DEVICES