MATERIALS SUITABLE TO SIMULATE SNOW DURING BREATHING EXPERIMENTS FOR AVALANCHE SURVIVAL RESEARCH
Authors:
Karel Roubík 1; Simon Walzel 1; Lenka Horáková 1; Alicia Refalo 2; Karel Sykora 3,4; Vaclav Ort 1; Ladislav Sieger 5
Authors place of work:
Department of Biomedical Technology, Faculty of Biomedical Engineering, Czech Technical University in Prague, Kladno, Czech Republic
1; École polytechnique universitaire de l'université Lyon-I, France
2; Department of Physiology and Biochemistry, Faculty of Physical Education and Sport, Charles University, Prague, Czech Republic
3; Military Department, Faculty of Physical Education and Sport, Charles University, Prague, Czech Republic
4; Department of Physics, Faculty of Electrical Engineering, Czech Technical University in Prague, Prague, Czech Republic
5
Published in the journal:
Lékař a technika - Clinician and Technology No. 1, 2020, 50, 32-39
Category:
Original research
doi:
https://doi.org/10.14311/CTJ.2020.1.05
Summary
Terrain experiments for avalanche survival research require appropriate snow conditions, which may not be available year round. To prepare these experiments and test the protocol, it might be advantageous to test them in a laboratory with a snow model. The aim of the study was to find a material that can be used to simulate snow for studying gas exchange of a person covered with avalanche snow. Three loose porous materials (perlite, wood shavings and polystyrene) were tested in two forms—dry and moisturized. Each volunteer underwent six phases of the experiment in random order (three materials, each dry and moisturized) during experimental breathing into the tested materials. Physiological parameters and fractions of oxygen and carbon dioxide in the airways were recorded continuously. All the materials selected as possible models of the avalanche snow negatively affected gas exchange during the breathing of the volunteers in a very similar extent. The time courses of the recorded parameters were very similar and were bordered from one side by the wet perlite and from the other side by the dry perlite. Therefore, other tested materials may be substituted with perlite with an appropriate water content. From all the tested materials, perlite is the best to simulate avalanche snow because of its homogeneity, reproducibility and easy manipulation.
Keywords:
avalanche snow – model – snow burial – survival – hypercapnia – breathing experiment
Introduction
Recently, the number of snow avalanche fatalities has increased. This is most likely related to an overall rise in backcountry utilization. Out-of-bounds skiers, ski patrollers, and backcountry skiers create the traditional group of avalanche victims [1]. Fatalities have increased recently among snowmobilers and snowboarders [2].
Investigation of all factors affecting survival of a vic-tim covered with avalanche snow is complicated during experiments with volunteers in the mountain terrain; therefore, isolated factors are often studied. As acute asphyxiation is the dominant cause of avalanche-related deaths [3–5], several experiments have been conducted so far [6–11] in order to study gas exchange processes separately. Traditionally, these breathing experiments with volunteers are organized in the mountain terrain with simulated avalanche snow. Nevertheless, design-ing, preparation and testing of the equipment and proto-col for these experiments are very time consuming and require conducting the tests with snow. This represents a significant obstacle and in countries not having required snow conditions all year round it limits the possibility of experimental research to a restricted peri-od of year. Therefore, a material simulating snow could facilitate the preparation and testing of breathing experi-ments before their implementation with real snow.
To our best knowledge, there has not been published a study dealing with materials that can simulate snow for the purpose of physiological breathing experiments. As the solubility of breathing gases in ice granules forming snow is negligible [12, 13], we do not suppose a reason-able interaction of the breathing gases with snow. Therefore, we hypothesize that a certain lose porous material would be suitable for these experiments. To support our hypothesis, Fig. 1 comprises curves of inspired and end-tidal fractions of oxygen and curves of inspired and end-tidal fractions of carbon dioxide when breathing into snow, wet and dry perlite. This experimental breathing was conducted by the main author of the current study prior designing of this study.
Even though the pilot experiment was not well controlled and recorded only in one subject, the curves corresponding to respiratory gases in snow are very similar and close to those recorded for wet and dry perlite. Their shapes appear to be very similar as well. Therefore, we hypothesize, that snow may be substituted with a solid stable material for gas exchange experi-ments.
For this study, we investigated several common mate-rials. The aim of the study was to find a material that might be used to simulate avalanche snow for studying gas exchange of a person covered with avalanche snow.
Methods
The prospective interventional randomized double-blind study has been conducted in the Snow research laboratory at the Faculty of Biomedical Engineering, Czech Technical University in Prague (FBME CTU). The study was approved by the Institutional Review Board of FBME CTU (Act No. A001/018). The study was registered in ClinicalTrials.gov database prior to its implementation (NCT03413878). All participants in-cluded in the study have signed an informed consent before their enrollment into the study.
Study group
Six volunteers (5M + 1F) of the age of 22.7 ± 0.9 were included into the study. The detailed demographic characteristics are presented in Table 1. Eligible partici-pants were volunteers from the Czech Army forces, studying at the Military Department of the Faculty of Physical Education and Sport, Charles University in Prague. All subjects were healthy and fit, classified according to the American Society of Anesthesiologists as ASA I [14].
The values are presented as mean ± standard deviation and range (minimum–maximum). Abreviations: BMI—Body Mass Index; FEV1—Forced Expiratory Volume in 1 second; FVC—Forced Vital Capacity.
The entrance examination, completed before the start of the study, comprised of these tests: electrocardio-graphy (Datex-Ohmeda S/5 monitor, Datex-Ohmeda, Madison, WI, USA), blood pressure (Datex-Ohmeda S/5 monitor, Datex-Ohmeda, Madison, WI, USA), spirometry (MSP1, Mesit, Uherske Hradiste, CZ), and assessment of the health conditions and family anamne-sis by a physician with a specialty in anesthesia and critical care. The exclusion criteria were Tiffeneau Index less than 0.70 and any cardiovascular or respira-tory condition.
Protocol
Each volunteer underwent six phases of the experi-ment: “PD”—breathing into dry perlite, “PW”—breathing into wet perlite, “RD”—breathing into dry polystyrene, “RW”—breathing into wet polystyrene, “HD”—breathing into dry wood shavings, “HW”—breathing into wet wood shavings. Volunteers were assigned to the PD, PW, RD, RW, HD and HW phase in a random order by throwing a dice. None the volun-teer, nor the examiner, knew the material of the current phase. At least a 4-hour recovery interval was inserted between two phases in each subject and only two phases per subject were possible during a day. All experiments were completed within three days.
Before starting the breathing experiment, sensors of a life function monitor were attached to the body of the subject. The following parameters were continuously monitored and recorded by Datex-Ohmeda S/5 monitor (Datex-Ohmeda, Madison, WI, USA): electrocardio-graphy (EKG), heart rate (HR), peripheral blood oxygen saturation (SpO2), inspiratory and end-tidal fractions of oxygen (FiO2, EtO2), inspiratory and end-tidal fractions of carbon dioxide (FiCO2, EtCO2), tidal volume (Vt), breathing frequency (BF), curves of airway pressure (Paw) and airflow (Qaw) measured in the airway opening, concentration of nitrous oxide (N2O) in the breathing circuit and non-invasive blood pressure (inter-mittently in one-minute intervals). The respiratory parameters as of Qaw, BF, Paw and Vt were measured using a respiratory sensor D-Lite (Datex-Ohmeda, Madison, WI, USA) connected between the mouthpiece and the Y-piece (Fig. 2). A spirometry D-Lite sensor also provided a gas sampling port for the DatexOhmeda S/5 monitor measuring FiO2, EtO2, FiCO2, EtCO2 and N2O concentration using an E-CAiOVX (Datex-Ohmeda, Madison, WI, USA) anesthesia and spirometry module. For safety reasons, another life function moni-tor Edan M3 (Edan Instruments, Nanshan Shenzhen, China) was used for parallel continuous SpO2 and HR measurements in the case that S/5 monitor did not show SpO2 or HR values.
The subject was placed in the prone position in front of a custom-made apparatus allowing fast exchange of the breathing media. The apparatus, depicted in Fig. 2, consisted of a stiff metal inverse cone, having the volume of 65 liters. The height of the cone was 1 m, the apex angle was 28 degrees. The inner wall of the cone was lined with a 1 mm thick polypropylene foil, which prevented freezing and heat conduction. At the bottom part of the cone a two-liter air pocket was created using a sieve placed perpendicularly to the cone axis at an appropriate position. The rest of the cone was filled with a tested material. The cone was filled 5 cm below the upper edge, which corresponds to a volume of 56 liters. After subtracting 2 liters of air pocket at the entrance to the cone, the total volume of tested material was 54 liters. A dust filter, a piece of corrugated tube 12 cm long, a flow sensor with a gas sampling port, a bacterial filter and a mouth piece were connected to the air pocket at the cone tip (see Fig. 2).
Before the breathing experiment, each subject was connected to the mouthpiece and the flow sensor only (not connected to the cone yet) allowing measurement of respiratory gasses and baseline ventilatory parame-ters. After reaching stable ventilatory parameters, espe-cially the steady tidal volume and breathing frequency (after approx. 5 minutes), the subject was connected to the cone and the breathing experiment was initiated. At the same time, nitrous oxide started to be administered to the vicinity of the head of the subject and the breathing circuit as a tracing gas for possible leak detection. Safety of the volunteers during the experi-ment was assured by advanced monitoring and con-tinuous evaluation of their physiological parameters. Furthermore, a clinician with a specialty in anesthesia and emergency medicine continuously assessed the state of the volunteer [15] and could terminate the experiment at any time.
The breathing experiment would be terminated due to one or more of the following reasons [15]: request of the volunteer; decision made by the anesthesiologist or the investigator testing the consciousness of the volunteer; end-tidal partial pressure of carbon dioxide (PaCO2) in the expired gas higher than or equal to 60 mm Hg [16]; presence of nitrous oxide in the breathing gas at any measurable concentration; or breathing in the material over 30 min. The experiments ended by disconnecting the mouthpiece from the breathing circuit so that the ventilatory parameters could be still measured. Another stabilization period (approx. 5 minutes) was inserted after the end of breathing trial. This phase lasted until steady readouts, similar to those recorded before the breathing experiment during the initial stabilization phase, were reached.
Tested materials
Three loose porous materials (Perlite, Wood shavings and Polystyrene) were used as a model of snow for breathing experiments. Each of the materials was used in two forms—dry and moisturized. Six materials were tested in total. The list of the materials and their basic properties are presented in Table 2. Their density was measured using a calibrated cylinder (1.6 L) and a weighing scale before every single experiment. The porosity of the material was determined by filling the calibrated cylinder full of the tested material with water and then weighted by the scale. Porosity was then calculated as:
porosity= 1-mmat-m0mtot-m0
,
where mmat is weight of the cylinder filled with the tested material, m0
is weight of the empty cylinder and mtot
is weight of the cylinder filled with the tested material and then flooded fully by water.
The perlite (abbreviated as P) is an industrial mineral and a commercial product useful for its low density after processing. The grain size of the perlite specified by the manufacturer was 1–3 mm (“Expandovaný perlit EP AGRO”; Perlit ltd., Šenov u N. Jičína, Czech Republic). The size of the wood shavings pieces (abbreviated as H) and their textures varied greatly from one to another from one to eight centimeters. The used polystyrene (abbreviated as R) was a hydrophobic expanded material with a typical grain size of 5 mm.
Data processing and statistics
The Kaplan Meier plot was used to analyze the time to breathing experiment termination involving all the volunteers and tested materials. For the next data analysis, the first 240 seconds of the experiment were analyzed. The following respiratory parameters were compared and statistically evaluated: SpO2, FiO2, EtO2, FiCO2, EtCO2. The statistical difference between the parameters were calculated in 15 second intervals from the beginning of the experiment (i.e., connecting the breathing circuit to the cone with the tested material). The statistical significance of the difference was tested using two-way ANOVA for repeated measures with LSD post-hoc tests (STATISTICA 7, StatSoft, Tulsa, OK, USA) after the Shapiro-Wilk data normality test. A value of p < 0.05 was considered statistically signifi-cant.
Results
All the six volunteers completed the whole protocol and were included in the data analysis. The Kaplan-Meier plot of the time to breathing experiment termina-tion for the whole group of six volunteers and all the tested materials was created and is presented in Fig. 3.
None of the experiments lasted longer than 390 sec-onds, and at the same time, none of the experiments was terminated earlier than after 240 seconds. For this rea-son, further data processing was performed using the first 240 seconds of the breathing experiments (i.e., 240 s since connecting the breathing circuit to the cone with the tested material).
There was not found any statistically significant dif-ference in SpO2 between any of the tested materials as demonstrated in Fig. 4. The biggest difference in SpO2 courses was measured between wet and dry perlites. Moisturizing had a much less effect in wood shavings and polystyrene than in perlite. In general, the decrease in SpO2 was faster in wet materials.
Inspiratory and end-tidal fractions of oxygen (Fig. 5) were the highest for the dry perlite and the lowest for the wet perlite from 60 seconds till 240 seconds. Curves of all other materials are situated between these two men-tioned materials. Statistically significant differences were found between dry perlite and wet perlite and be-tween dry perlite and wet polystyrene from 120 seconds for FiO2 and from 180 seconds for EtO2.
In the inspiratory and end-tidal fractions of carbon dioxide curves, there was not found any statistically significant difference between any of the tested mate-rials. The curves of the inspiratory and end-tidal frac-tions of carbon dioxide are presented in Fig. 6.
Discussion
The main finding of the study is that all the tested materials exhibited very similar effect on breathing gasses. Except for the differences in inspiratory and end-tidal fractions of oxygen, all the measured courses were very similar. From the measured data, the materials seemed to be interchangeable i.e. having a very similar effect on the breathing gasses and duration of the experi-ments. Even though the differences are very small, it seems that various water content in the tested materials had stronger effect than materials themselves.
According to all the measured curves (Fig. 4–6), the dry perlite represents the material that affects gas ex-change less than all the other materials in their wet and dry forms. On the other hand, wet perlite compromises the gas exchange more than all the other tested mate-rials.
Therefore, by changing water content in perlite, any other material can be mimicked. When working with the three tested materials, certain differences should be mentioned, that may negatively affect the possible ex-periments when these materials should serve as models of avalanche snow. First, the density of materials can be changed by their compression. The most prone to compression induced changes in density were the wood shavings (both wet and dry), then polystyrene. Further-more, polystyrene does not absorb water well and as a result, preparing polystyrene with a predefined water content may be tricky. The most resistant material to compression was perlite in both its forms. Therefore, the perlite represents a material with the most repro-ducible physical properties and it can be expected that repeated preparation of perlite environment for breath-ing experiments will be associated with the lowest variations.
During the experiment, a two-liter air pocket was present between volunteer and the tested materials. The presence of air pocket or its size were selected as a typical configuration of already published experi-mental studies [8, 9, 15].
In the current study, the group of volunteers con-sisted of well trained and healthy military profess-sionals. This constitution of the study group assured less possible interindividual differences.
The current study did not intend to mimic the real conditions when a person breathes in the avalanche snow. Rather, the study was designed to investigate differences between various materials that can be used as a physical model of snow. For this reason, a special apparatus was designed in the form of an inverse cone. Using this apparatus, the tested materials can be ex-changed easily and promptly and conditions of mea-surement including geometry are standardized.
The study has several limitations. Firstly, the study group consisted of only six volunteers. We do not suppose this feature as a significant limitation because the aim of this study was not to precisely characterize properties of the individual tested materials. The aim of the study was to compare these materials and to find out whether the materials are almost interchangeable or whether they affect breathing differently. This study serves as a pilot experiment; the results of this study will be used for a design of a standard research study involving real snow as well. For these reasons and considering the relative homogeneity of the group men-tioned above, the number of participants is sufficient.
Secondly, only first 240 seconds of each breathing trial was evaluated because 240 seconds is the time for which all the volunteers were able to sustain breathing under compromised conditions. Nevertheless, we sup-pose this time as sufficient for analyzing effects of individual materials. This represents a difference from other published studies [7–10] aimed at investigation of lengths of survival and associated effects.
Conclusion
Three materials (perlite, wood shavings and polysty-rene), selected as possible models of the avalanche snow, negatively affected gas exchange during breath-ing of the volunteers in a similar extent. The time courses of the recorded parameters were very similar and were bordered from one side by the wet perlite and from the other side by the dry perlite. The biggest difference reached by moisturizing was found between dry and wet perlite. From all the tested materials, perlite is the best to simulate avalanche snow because of its homogeneity, reproducibility and easy manipu-lation. By changing water content in perlite, it is possi-ble to modify the properties affecting gas exchange and the other tested materials can be substituted by perlite with an appropriate water content. A further study is needed to compare perlite and real avalanche snow during breathing experiments.
Acknowledgement
The study was supported by Czech Technical University Grant No. SGS20/202/OHK4/3T/17
Prof. Ing. Karel Roubík, Ph.D.
Department of Biomedical Technology
Faculty of Biomedical Engineering
Czech Technical University in Prague
nám. Sítná 3105, CZ-272 01 Kladno
E-mail: roubik@fbmi.cvut.cz
Phone: +420 603 479 901
Zdroje
- Page CH, Atkins D, Shockley L, Yaron M. Avalanche deaths in the United States: a 45-year analysis. Wilderness and Environ-mental Medicine. 1999;10:146–51.
- DOI: 10.1580/1080-6032(1999)010[0146:aditus]2.3.co;2
- Jekich BM, Drake BD, Nacht JY, Nichols A, Ginde AA, Davis CB. Avalanche fatalities in the United States: a change in demo-graphics. Wilderness & environmental medicine. 2016 Mar 1; 27(1):46–52. DOI: 10.1016/j.wem.2015.11.004
- Boyd J, Haegeli P, Abu-Laban RB, Butt JC. Patterns of death among avalanche fatalities: a 21-year review. Canadian Medical Association Journal. 2009;180(5):507–12. DOI:10.1503/cmaj.081327
- McIntosh SE, Grisom CK, Olivares CR, Tremper B. Cause of death in avalanche fatalities. Wilderness & Enviromental Medicine. 2007;18(4):293–7.
- DOI: 10.1580/07-WEME-OR-092R1.1
- Hohlrieder M, Brugger H, Schubert HM, Mair P. Pattern and severity of injury in avalanche victims. High Altitude Medicine & Biology. 2007;8(1):56–61. DOI: 10.1089/ham.2006.0815
- Falk M, Brugger H, Adler-Kastner L. Avalanche survival chances. Nature. 1994;368:21. DOI: 10.1038/368021a0
- Grissom CK, Radwin MI, Harmston CH, Hirshberg EL, Crowley TJ. Respiration during snow burial using an artificial air pocket. Jama. 2000;283(17):2261–71. DOI: 10.1001/jama.283.17.2266
- Paal P, Strapazzon G, Braun P, Ellmauer PP, Schroeder DC, Suman G, et al. Factors affecting survival from avalanche burial–A randomised prospective porcine pilot study. Resuscitation. 2013;84(2):239–43. DOI: 10.1016/j.resuscitation.2012.06.019
- Brugger H, Sumann G, Meister R, Adler-Kastner L, Mair P, Gunga HC. Hypoxia and hypercapnia during respiration into an artificial air pocket in the snow: implications for avalanche survival. Rescuscitation. 2003;58:81–8.
- DOI: 10.1016/s0300-9572(03)00113-8
- Strapazzon G, Paal P, Schweizer J, Falk M, Reuter B, Schenk K, Gatterer H, Grasegger K, Dal Cappello T, Malacrida S, Riess L. Effects of snow properties on humans breathing into an artificial air pocket–an experimental field study. Scientific reports. 2017; 7(1):17675. DOI: 10.1038/s41598-017-17960-4
- Radwin IM, Grisom CK, Scholand MB, Harmston CH. Normal Oxygenetation and Ventilation during Snow Burial by the Exclusion of Exhaled Carbon Dioxine. Wilderness Environ-mental & Medicine. 2001;12:256–62.
- DOI: 10.1580/1080-6032(2001)012[0256:noavds]2.0.co;2
- Ahn J, Headly M, Wahlen M, Brook EJ, Mayewski PA, Taylor KC. CO2 diffusion in polar ice: observations from naturally formed CO2 spikes in the Siple Dome (Antarctica) ice core. Journal of Glaciology [online]. 2008;54(187):685–95.
- DOI: 10.3189/002214308786570764
- Hemmingsen E. Permeation of Gases through Ice. Institute of Zoophysiology, University of Oslo (1958). Tellus 11, 355–9. DOI: 10.1111/j.2153-3490.1959.tb00041.x
- American Society of Anesthesiologists, INC. New Classifi-cation of Physical Status, Anesthesiology, 1963; 24: 111.
- Roubík K, Sieger L, Sykora K. Work of Breathing into Snow in the Presence versus Absence of an Artificial Air Pocket Affects Hypoxia and Hypercapnia of a Victim Covered with Avalanche Snow: A Randomized Double Blind Crossover Study. PLoS ONE. 2015;10(12):e0144332.
- DOI: 10.1371/journal.pone.0144332
- Lumb AB. Nunn's Applied Respiratory Physiology. 7th edition. Elsevier, 2012.
Štítky
BiomedicínaČlánok vyšiel v časopise
Lékař a technika
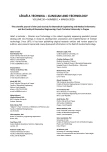
2020 Číslo 1
Najčítanejšie v tomto čísle
- VERIFICATION OF CLINICAL ACCURACY OF AUTOMATED NON-INVASIVE SPHYGMOMANOMETERS: IS IT APPROPRIATE TO USE BLOOD PRESSURE SIMULATORS?
- INFLUENCE OF THE USE OF GRAVITY SETS IN A PRESSURE VOLUMETRIC INFUSION PUMP WITH AN IMPACT ON THE ACCURACY OF INFUSION SOLUTION FLOWS
- FUNCTIONALIZATION OF POLYMERIC NANOFIBERS USING PLATELETS FOR MELANOCYTE CULTURE
- MATERIALS SUITABLE TO SIMULATE SNOW DURING BREATHING EXPERIMENTS FOR AVALANCHE SURVIVAL RESEARCH