MICROBIOME AND UVEITIDES. A REVIEW
Authors:
M. Brichová 1; P. Svozílková 1; A. Klímová 1; O. Dušek 1; M. Kverka 2; prof. MUDr. Jarmila Heissigerová, Ph.D., MBA 1
Authors place of work:
Oční klinika, 1. lékařská fakulta, Univerzita Karlova a Všeobecná fakultní nemocnice v Praze
1; Mikrobiologický ústav AVČR, Praha
2
Published in the journal:
Čes. a slov. Oftal., 78, 2022, No. 2, p. 47-52
Category:
doi:
https://doi.org/10.31348/2021/30
Summary
Microorganisms inhabiting all surfaces of mucous membranes and skin and forming a complex ecosystem with the host is called microbiota. The term microbiome is used for the aggregate genome of microbiota. The microbiota plays important role in the mechanisms of number of physiological and pathological processes, especially of the host’s immune system. The origin and course of autoimmune diseases not only of the digestive tract, but also of the distant organs, including the eye, are significantly influenced by intestinal microbiota.
The role of microbiota and its changes (dysbiosis) in the etiopathogenesis of uveitis has so far been studied mainly in experimental models. Reduction of severity of non-infectious intraocular inflammation in germ-free mice or in conventional mice treated with broad-spectrum antibiotics was observed in both the induced experimental autoimmune uveitis model (EAU) and the spontaneous R161H model. Studies have confirmed that autoreactive T cell activation occurs in the intestinal wall in the absence of retinal antigen. Recent experiments focused on the effect of probiotic administration on the composition of intestinal microbiota and on the course of autoimmune uveitis. Our study group demonstrated significant prophylactic effect of the administration of the probiotic Escherichia coli Nissle 1917 on the intensity of inflammation in EAU.
To date, only a few studies have been published investigating intestinal dysbiosis in patients with uveitis (e.g., in Behcet’s disease or Vogt-Koyanagi-Harada syndrome). The results of preclinical studies will be presumably used in clinical practice, mainly in the sense of prophylaxis and therapy, such as change in the lifestyle, diet and especially the therapeutic use of probiotics or the transfer of faecal microbiota.
Keywords:
Microbiome – Uveitis – microbiota – probiotics
INTRODUCTION
Many medical fields, including Ophthalmology, are taking a new interest in the human microbiome. Microbiome dysregulation has been observed in a number of autoimmune and autoinflammatory disorders, including inflammatory bowel disease [1,2,3,4,5,6], allergies [7,8,9], eating disorders [10] and many other illnesses, including uveitis [11,12].
Whether the microbiome influences the course or even the prevention of intraocular inflammation (uveitis) is a question that has not yet been satisfactorily resolved and remains a subject of research. Uveitis is a sight-threatening disease that accounts for at least 10 % of all cases of blindness in developed countries [13,14]. Less than 30 % of uveitides can be identified by an infectious cause. In such cases, targeted therapy is indicated by pathogen identification (PCR, serology) or by the characteristic clinical picture. Most uveitides are non-infectious and belong to the immune-mediated inflammatory disease group (IMID) [15]. Approximately 25 % of non-infectious intraocular inflammations are part of some systemic disease (e.g. ankylosing spondyloarthritis, juvenile idiopathic arthritis, sarcoidosis, multiple sclerosis, Behcet's disease). If uveitis activity threatens the visual function of the eye, the use of general therapy is indicated. The choice of medication depends on several factors – the type of uveitis, the age and health of the patient, and the possible presence of any associated systemic diseases. Corticosteroids are usually the first method of choice, followed by corticosteroids sparing immunosuppressive and biological therapy. In many patients, the treatment of intraocular inflammation is limited by the efficacy of the preparation, the patient’s toleration of the drug, and the potential for the development of side effects [16]. In uveitis, as in other diseases in the IMID group, the causes of its development and new therapeutic strategies are still being investigated. One of the directions of research is the issue of the microbiome in the pathogenesis of IMID, and the possibility of its influence in the therapy of these diseases [17,18].
Microbiota
The microbiota inhabits all surfaces of the body – skin, conjunctiva, and the mucous membranes of the respiratory, urogenital, and digestive tracts. The microbiota of the large intestine is the most numerous (1012/g content). Table. In humans, the intestinal microbiota forms a complex ecosystem, consisting of more than 1 000 species of bacteria, yet it also contains Archaea, viruses and microscopic fungi and parasites [20,21,22]. Specific properties of the digestive tract predispose it to colonisation by microbes of several basic strains – Firmicutes (especially the Clostridia and Bacilli classes), Bacteroidetes (especially the families Bacteroides and Prevotella), Actinobacteria, and Proteobacteria [23]. This typical composition, under the influence of environmental factors, stabilises during the first three years of life. [18,24]. About 60 % of the microbes are specific to each human [20]. Although the major components of the intestinal microbiome remain relatively stable during adulthood, long-term dietary changes or antibiotics can significantly affect the microbiome, and ill-advised interventions in the microbiome composition can have health consequences [25,26].
Although interest in the intestinal microbiome began more than 100 years ago, methods previously used for microbiome analysis (microscopic, cultural, and biochemical) have had considerably limited success. Up to 70 % of intestinal bacteria cannot be cultivated by conventional microbiological methods. The development of molecular biological and sequencing techniques in the 1990s made it possible to read the human genome in 2000 [27]. Ten years later, with great effort, the human microbiome, which is about 150 times the size of the human genome and is sometimes called the "second human genome", was analysed [20]. Only the development of molecular biological analysis methods has significantly improved our knowledge about the composition of the microbiome. Metagenomics uses the methods of sequencing some specific microbial genes, such as the bacterial 16S rRNA gene. The gene is composed of evolutionarily stable sections of DNA and from variable sections, which make it possible to identify a specific microbe by comparing these sequences with a database [12,20,22]. Gnotobiotic studies are important in investigating the importance of microbiota. These make it possible to monitor the influence of defined microbes (colonisation study), or the effect of the absence of microbes (germ-free study) on the development of physiological functions and pathological processes in experimental animal models [22, 28,29].
Importance of microbiota for hosts
The microbiota plays an important role in a number of biological processes, both directly, through the production of bioactive molecules, and indirectly, by influencing the development and reactivity of the host’s epithelial or immune cells. During life, microbes interact with the immune system in a way that maintains the body's defences, as well as the body’s tolerance of commensal microbes and other harmful substances from the environment. The increase in the body's defences due to microbes is called colonisation resistance. This can be straightforward, with commensal microbes competing with pathogens for nutrients and access to the body's surface, or killing them by producing toxins. Indirect colonisation resistance is caused by influencing the development of the immune system and by stimulating the cells present near body surfaces. The intestinal microbiota affects the metabolic processes of the host, the efficiency of nutrient absorption, performs the cleavage of indigestible polysaccharides while forming short-chain fatty acids, and produces some vitamins [22]. An unfavourable change in the composition or function of the microbiota, known as dysbiosis, is present in a number of pro-inflammatory, autoimmune, and cancerous diseases. The most common form of dysbiosis is the loss of variation (decrease in diversity) of microbiota, which is typical of our lifestyle in an industrialised society, and which could explain the higher incidence of these diseases in developed countries [30]. It should be noted that, compared to people living in primitive conditions, the diversity of intestinal microbiotas of healthy people in developed countries is low and that this change during industrialisation has reduced the ability to defend against pathogens and vitamin production, but has improved, for instance, carbohydrate and xenobiotic metabolism [31,32,33].
Interaction of microbiota with the immune system
The microbiota influences the host organism both through the production of bioactive molecules (e.g. short-chain fatty acids – propionate, butyrate, acetate) and through the modulation of cellular and humoral immunity. The main mediators of the immune response are intestinal epithelial cells (forming an area of 200 m2) and leukocytes. Surface receptors for pathogenic microbes are located on the basolateral membrane of epithelial cells. After recognition of the pathogen by these cells, their immune mechanisms are activated and triggered (cytokine production, induction of autophagy and production of antimicrobial peptides). Under the influence of cytokines, dendritic cells are activated in the intestinal mucosa, which look for microbes and move them to the lymph nodes, where they elicit an adaptive immune response. The released cytokines, such as interferon gamma (IFNγ), tumour necrosis factor alpha (TNFα), increase the permeability of the intestinal wall, thereby exacerbating inflammation. The immune response also affects microbes through their metabolites. For example, short-chain fatty acids promote regulatory T-cell proliferation (Treg) and the production of anti- inflammatory interleukin 10 (IL10). At the same time, however, there is no significant immunosuppression or risk of infection, as these anti-inflammatory changes are accompanied by an increase in the microbicidal activity of macrophages [34]. The presence of segmented filamentous bacteria (Clostrideacea spp.) in the intestinal microbiota induces the formation of helper T lymphocytes 17 (Th17) and subsequently IL17 and IL22, which protect the intestine from infection by extracellular fungi and bacteria. In contrast, in the autoimmune processes, Th17, IL17 and IL22 have an inflammatory effect. A particularly important part of the stability of the intestinal wall is the secretory IgA (S-IgA) produced by plasma cells. S-IgA activity is regulated by follicular regulatory T cells (Tfr), i.e. specialized Treg in the germinal centres of Peyer's patches, and is directed against pathobionts [35,36,37,38,39,40]. In the development of idiopathic intestinal inflammation, the influence of commensal bacteria or dysbiosis in an individual with genetic predispositions and in interaction with environmental factors is indisputable [12,3,4,5,6,41]. However, the intestinal microbiota influences immune processes not only in the gastrointestinal tract, but also in distant organs and tissues through the above-mentioned mechanism. Therefore, the role of microbiota is intensively studied for its possible connections with obesity [42], allergies [7,8,9], diabetes mellitus [43,44], rheumatic diseases [45], as well as multiple sclerosis, neurodegenerations and mental illnesses [46,47,48]. Dysbiosis usually increases the severity of induced inflammation in most animal models (idiopathic intestinal inflammation, multiple sclerosis, arthritis, spondyloarthritis, etc.), but decreases the severity in models of type 1 diabetes mellitus [49].
Unlike the human genome, the microbiome can be affected. The composition of the microbiome, and thus its effect on the host organism, can be modified mainly through diet and lifestyle [50]. Protein and animal fats create conditions for the growth of Bacteroides, while a diet with a predominance of polysaccharides favours the growth of the Prevotella strain. Currently, the administration of probiotics, prebiotics, or synbiotics is most often used to influence the microbiome. Probiotic cultures are present in some foods (cheese, yogurt) and are used in the form of food supplements or drugs. These are lactic acid bacteria (lactobacilli, bifidobacteria), enterococci (some strains of Escherichia coli) or yeast [51]. Prebiotics stimulate the growth of beneficial probiotic bacteria. Synbiotics are a combination of probiotics and prebiotics. Some probiotic bacteria have immunomodulatory effects, others have anti-inflammatory effects. Functionally defined probiotics should be used for individual diseases. One probiotic, e.g. E. coli O83, can have different effects on different diseases – positive for allergies, negative for intraocular inflammation [8, 52]. Probiotics mainly have a preventive effect and their therapeutic use is still considered to be limited (e.g. for diarrhoea in antibiotic treatment, for rotavirus diarrhoea, irritable bowel syndrome and ulcerative colitis) [12,53,4]. Currently, research is focused on the possibility of the preparation and application of recombinant strains of bacteria that produce biologically active molecules, such as cytokines with an anti-inflammatory activity [12]. Antibiotics can also have a significant effect on the microbiome, and more seriously when given in early childhood [12,55,56,57]. Faecal bacteriotherapy (i.e. transfer of intestinal microbiota from healthy donors) to influence dysbiosis is used mainly in Clostridial colitis [12,58]. The potential of this method in ulcerative colitis, irritable bowel syndrome, type 2 diabetes mellitus, obesity, liver encephalopathy and some mental illnesses is being investigated [12,59].
Microbiome and uveitis
Autoimmune uveitis is a heterogeneous group of T-cell mediated diseases, the pathogenesis of which, however, has not yet been satisfactorily clarified. It is thought that, due to infectious agents as triggers of autoimmune diseases in genetically predisposed individuals, there may be confusion between foreign antigens and autoantigens due to the similarity of their epitopes [60]. The influence of infection in the development of autoimmune diseases is confirmed through the method of induction of these diseases in experimental animal models, including the classic model of experimental autoimmune uveitis (EAU). In this method, in addition to autoantigens, components of infectious agents (adjuvants) are also used, which are necessary for the stimulation of cells of the innate immune system. In the EAU model, intraocular inflammation is induced by subcutaneous application of an intraretinal retinol-binding protein (IRBP) in a complete Freund's adjuvant (deceased mycobacteria), which is potentiated by a pertussis toxin [61]. The introduction of the EAU model was crucial for research of proinflammatory antigen-specific T cells (Th1, Th17), antigen presenting dendritic cells, macrophages and other myeloid cells, proinflammatory cytokines (TNFα, IL1, IL12, IL23) and Treg [62,63]. For a number of research projects in uveitis, i.e. inflammation in an immune privileged organ, the R161H mouse model of spontaneous uveitis was developed. This study is one in which the subject does not undergo antigen induction (IRBP), as the peripheral immune system at a functional blood-eye barrier is not confronted with this antigen. Using this model, Horai et al. found that the reduction of intestinal microbiota by broad-spectrum antibiotics (mixture of ampicillin, metronidazole, neomycin and vancomycin) significantly reduced the activity of spontaneous uveitis. Subsequently, they described the same antibiotics for the same model in germ-free conditions. In both models, they observed a decrease in Th17 in the lamina propria of the intestinal wall. They thus confirmed that the activation of autoreactive T lymphocytes against retinal antigen takes place in the intestinal wall in the absence of retinal antigen and is dependent on commensal intestinal bacteria [64]. Heissigerova et al. compared the severity of EAU in non- -microbial mice, conventional mice, and conventional mice treated with orally administered broad-spectrum antibiotics (metronidazole and ciprofloxacin), with treatment initiation from the day of induction or 1 week before induction, biomicroscopically, histologically, and immunohistologically. In non-microbial and conventional mice treated one week before induction, the study showed a statistically significant reduction in EAU intensity. In the retina of germ-free mice, compared to conventionally bred mice, lower infiltration by macrophages and T lymphocytes, as well as a decrease in proinflammatory cytokines (IFNγ, IL17) and an increase in Treg in the cervical nodes were observed [65]. Seidler Stangova et al. further analysed these data and recognised that metronidazole monotherapy given orally one or two weeks prior to EAU induction significantly reduced the intensity of inflammation [66]. All these results provide evidence for the importance of the role of microbiota in the pathogenesis of autoimmune uveitis. Similar results were published by Nakamura et al. In their study, they described a reduction in the clinical activity of inflammation in EAU by oral application of vancomycin or metronidazole (initiated 1 week before induction), which was expected to have a direct effect on the intestinal microbiota. This effect was not observed in subjects who underwent intraperitoneal administration of the above antibiotics or with ampicillin or neomycin. In the group with effective antibiotic therapy, they also first experienced an increase in Treg in the lamina propria of the intestinal mucosa, then in the mesenteric and cervical lymph nodes and in the retina. Against the untreated control group, the authors demonstrated a lower proportion of proinflammatory cytokines (IFNγ, TNFα, IL2, IL17) in the cervical nodes of the treated group [67]. There are studies which document the protective effect of intestinal microbiota on immunological processes in the eye [68] and confirm that dysbiosis induces a pro-inflammatory state in the host [37]. The procedures described above are a form of microbiota depletion or reduction (germ-free models, antibiotic treatment). Another possible approach is to induce a change in the composition of the intestinal microbiota by administering short-chain fatty acids that have an immunomodulatory effect (reduction of pathogenic Th17 in favour of Treg), enhance barrier function and prevent lymphocyte migration between the gastrointestinal tract and the eye [69,70].
Another way to affect the microbiome is to enrich the microbiota with probiotics. Kim et al. observed in the EAU model, after 3 weeks of probiotic gavage (Lactobacillus casei, Lactobacillus acidophilus, Lactobacillus reuteri, Bifidobacterium bifidum, and Streptococcus thermophilus), a decrease in the severity of intraocular inflammation in histological sections, an increase in Treg, and a depletion of IL17, IFNγ and cytotoxic T cells in the cervical lymph nodes [71].
Dusek et al. documented the protective effect of live Escherichia coli Nissle 1917 in a regimen before or simultaneously with EAU induction. Conversely, the use of E. coli O83: K24: H31 led to increased inflammatory activity. Deceased (autoclaved) probiotics had no protective effect in any regimen. Similarly, live E. coli Nissle 1917, whose therapeutic application was started 2 weeks after the induction of uveitis, had no protective effect. The reduction or prevention of the development of inflammation was most probably mediated by a change in the reactivity of the immune system, with a consequent decrease in inflammation activity. This study showed that the reduction in inflammatory reactivity to the IRBP antigen occurred at the site of its presentation (inguinal node). Another significant effect on reducing inflammation may have been influencing the type of macrophages involved in the inflammatory process and enhancing intestinal mucosal integrity. The results of the project suggested that the prevention of ocular inflammation with the use of given probiotics is more successful than its subsequent treatment, and that manipulation of intestinal microbes could be a rational therapeutic approach [52].
To date, only a few observational studies comparing the intestinal microbiota in patients with uveitis and healthy control groups have been published. Intestinal dysbiosis has been reported in patients with Behcet's disease when compared to healthy control groups [72]. In contrast, the work of Huang et al. did not confirm a significant difference in the composition of the intestinal microbiota between patients with anterior uveitis and control groups. However, the authors noted a statistically significant difference in the analysis of stool metabolites. They detected elevated levels of linoleic acid, azelaic acid and inosine in patients with anterior uveitis [73]. Kalyana Chakravarthy et al. showed in patients with uveitis, in contrast to a healthy control group, a decrease in the diversity of intestinal microbiota, including anti-inflammatory microbes (Faecalibacterium, Bacteroides, Lachnospira, Ruminococcus) and an increase in pro-inflammatory (Prevotella) and pathogenic microflora (Streptococcus) [74]. The same study group published work on reducing yeast diversity in patients with uveitis [75]. Ye et al. did not record a significant difference in the composition of the intestinal microbiota between patients with Vogt-Koyanagi-Harad (VKH) syndrome and healthy control groups. However, they report a relative increase in Paraprevotella spp. in patients with uveitis and a reduction in the relative proportion of Clostridium spp., Bifidobacterium spp., Candidatus Methanomethylophilus alvus and Methanoculleus spp. [76].
Research into the effect of stool transplants on microbiota in intraocular inflammation is still in its infancy. Ye et al. transplanted the stools of patients with VKH syndrome in antibiotic treated B10RIII mice prior to EAU induction. In these mice, they experienced a significantly more severe course of EAU than in mice after stool transplantation from healthy individuals. The same results were seen in a similar study, using the stool of patients with Behcet's disease [76,77].
CONCLUSION
Intestinal microbiota certainly affects many physiological and pathological processes, not only in the intestine, but also in distant organs. This extraintestinal effect is mediated by its interaction with the immune system. In the case of uveitis, the importance of intestinal microbiota in their aetiopathogenesis is documented primarily in experimental models. The results of published studies suggest that the severity of autoimmune uveitis could be alleviated by probiotic manipulation of the intestinal microbiota. However, it is necessary to develop a deeper understanding of the interaction of microbes with the immune system of patients with uveitis and to verify these findings through a clinical study in order to utilise this method.
The authors of the study declare that no conflict of interest exists in the compilation, theme and subsequent publication of this professional communication, and that it is not supported by any pharmaceutical company. The study has not been submitted to any other journal nor has it been printed elsewhere.
Received: 5 March 2021
Accepted: 29 March 2021
Accepted on-line: 25 November 2021
MUDr. Michaela Brichová
Oční klinika 1. LF UK a VFN
U nemocnice 2
128 08 Praha 2
E-mail: michaela.brichova@vfn.cz
Zdroje
1. Butto LF, Schaubeck M, Haller D. Mechanisms of microbe-host interaction in Crohn‘s disease: dysbiosis vs. pathobiont selection. Front Immunol. 2015;6:555. Available from:
2. Sokol H, Seksik P, Furet JP, et al. Low counts of Faecalibacterium prausnitzii in colitis microbiota. Inflamm Bowel Dis. 2009;15(8):1183-1189.
3. Powrie F, Leach MW. Genetic and spontaneous models of inflammatory bowel disease in rodents:evidence for abnormalities in mucosal immune regulation. Ther Immunol. 1995;2(2):115- 123.
4. Hudcovic T, Stepankova R, Cebra J, et al. The role of microflora in the development of intestinal inflammation: Acute and chronic colitis induced by dextran sulphate in germ-free and conventionally reared immunocompetent and immunodeficient mice. Folia Microbiol. 2001;46(6):565-572.
5. Stepankova R, Powrie F, Kofronova O, et al. Segmented filamentous bacteria in a defined bacterial cocktail induce intestinal inflammation in SCID mice reconstituted with CD45RBhigh CD4+T cells. Inflamm Bowel Dis. 2007;13(10):1202-1211.
6. Bajer L, Kverka M, Kostovcik M, et al. Distinct gut microbiota profiles in patients with primary sclerosing cholangitis and ulcerative colitis. World J Gastroenterol. 2017;23(25):4548-4558.
7. Tlaskalová-Hogenová H, Městecky J. Účast slizničního imunitního systému a komenzálních bakterií v alergii. Alergie. 2012;14(2):124- 133. Czech.
8. Hrdy J, Vlasakova K, Cerny V, et al. Decreased allergy incidence in children supplemented with E. coli O83:K24:H31 and its possible modes of action. Eur J Immunol. 2018;48(12):2015-2030.
9. Bunyavanich S, Berin MC. Food allergy and the microbiome: Current understandings and future directions. J Allergy Clin Immunol. 2019;144(6):1468-1477.
10. Roubalova R, Prochazkova P, Papezova H, et al. Anorexia nervosa: Gut microbiota-immune-brain interactions. Clin Nutr. 2020;39(3):676-684.
11. Mölzer C, Heissigerova J, Wilson HM, Kuffova L, Forrester JV. Immune privilege: The microbiome and uveitis. Front Immunol. 2021;11:608377.
12. Tlaskalová-Hogenová H, Jirasková Zákostelská Z, Petanová J, Kverka M. Mikrobiota, imunita a imunologicky mediované choroby. Vnitr Lek. 2019;65(2):98-107. Czech.
13. Rothova A, Suttorp-van Schulten MS, Frits Treffers W, Kijlstra A. Causes and frequency of blindness in patients with intraocular inflammatory disease. Br J Ophthalmol. 1996;80(4):332-336.
14. Suttorp-Schulten MS, Rothova A. The possible impact of uveitis in blindness: a literature survey. Br J Ophthalmol. 1996;80(9):844-848.
15. Klímová A, Brichová M, Říhová E, Heissigerová J, Svozílková P.Immune-mediated intraocular inflammation. Cesk Slov Oftalmol. 2021. Ahead of print. doi: 10.31348/2021/7
16. Jabs DA. Immunosuppression for the uveitides. Ophthalmology. 2018;125(2):193–202.
17. Kodati S, Sen HN. Uveitis and the gut microbiota. Best Pract Res Clin Rheumatol. 2019;33(6):101500.
18. Young VB. The role of the microbiome in human health and disease: an introduction for clinicians. BMJ 2017; 356: j831. Available from:
19. Sender R, Fuchs S, Milo R. Revised Estimates for the Number of Human and Bacteria Cells in the Body. PLoS Biol. 2016;14(8):e1002533.
20. Qin J, Li R, Raes J, et al. A human gut microbial gene catalogue established by metagenomic sequencing. Nature. 2010;464(7285):59-65.
21. Hooper LV, Gordon JI. Commensal host-bacterial relationships in the gut. Science. 2001;292(5519):1115-1118.
22. Kverka M, Tlaskalova-Hogenova H. Intestinal Microbiota: Facts and Fiction. Dig Dis. 2017;35:139-147.
23. Bajer L, Kverka M, Kostovcik M, et al. Distinct gut microbiota profiles in patients with primary sclerosing cholangitis and ulcerative colitis. World J Gastroenterol. 2017 7;23(25):4548-4558.
24. Yatsunenko T, Rey FE, Manary MJ, et al. Human gut microbiome viewed across age and geography. Nature 2012; 486(7402):222-227.
25. David LA, Materna AC, Friedman J, et al. Host lifestyle affects human microbiota on daily timescales. Genome Biol 2014;15(7):R89.
26. Lange K, Buerger M, Stallmach A, Bruns T. Effects of Antibiotics on Gut Microbiota. Dig Dis 2016;34(3):260-268.
27. International Human Genome Sequencing Consortium. Initial sequencing and analysis of the human genome. Nature. 2001;409:860-921.
28. Tlaskalova-Hogenova H, Kverka M, Verdu EF, et al. Chapter 8 – Genotobiology and the study of complex interactions between the intestinal microbiota, probiotics, and the host. In: Mestecky J, Strober W, Russell MW et al (eds). Mucosal Immunology (4th ed). Academic Press: Boston 2015:109-133. ISBN 978-0124158474.
29. Falk PG, Hooper LV, Midtvedt T, et al. Creating and maintaining the gastrointestinal ecosystem: What we know and need to know from gnotobiology. Microbiol Mol Biol Rev. 1998;62(4):1157-1170.
30. Abrahamsson TR, Jakobsson HE, Andersson AF, Björkstén B, Engstrand L, Jenmalm MC. Low diversity of gut microbiota in infants with atopic eczema. J Allergy Clin Immunol. 2012;129:434-440.
31. Rampelli S, Schnorr SL, Consolandi C, et al. Metagenome sequencing of the Handza hunter- gutherer gut microbiota. Curr Biol. 2015;25:1682-1693.
32. Gomez A, Petrzelkova KJ, Burns MB, et al. Gut microbiome in coexisting BaAka Pygmies and Bantu reflects gradients of traditional subsistence patterns. Cell Rep. 2016;14:2142-2153.
33. Mancabelli L, Milani C, Andrea Lugli GA, et al. Meta-analysis of human gut microbiome from urbanized and pre-agriculture population. Environ Microbiol. 2017;19:1379-1390.
34. Schulthess J, Pandey S, Capitani M, et al. The short chain fatty acid butyrate imprints an antimicrobial program in macrophages. Immunity. 2019;50(2):432-445.
35. Xie MM, Dent AL. Unexpected Help: Follicular regulatory T cells in the germinal center. Front Immunol. 2018;9:1536.
36. Palm NW, de Zoete MR, Cullen TW, et al. Immunoglobulin A coating identifies colitogenic bacteria in inflammatory bowel disease. Cell. 2014;158(5):1000-1010.
37. Tlaskalova-Hogenova H, Stepankova R, Kozakova H, et al. The role of gut microbiota (commensal bacteria) and the mucosal barrier in the pathogenesis of inflammatory and autoimmune diseases and cancer: Contribution of germ-free and gnotobiotic animal models of human diseases. Cell. Mol. Immunol. 2011;8:110-120.
38. Alexander KL, Targan SR, Elson CO. Microbiota activation and regulation of innate and adaptive immunity. Immunol Rev. 2014;260(1):206-220.
39. Belkaid Y, Hand TW. Role of the microbiota in immunity and inflammation. Cell. 2014;157(1):121-141.
40. Smith PM, Howitt MR, Panikov N, et al. The microbial metabolites, short-chain fatty acids, regulate colonic Treg cell homeostasis. Science. 2013;341(6145):569-573.
41. Hudcovic T, Stepankova R, Cebra J, H. Tlaskalova-Hogenova H. The role of microflora in the development of intestinal inflammation: acute and chronic colitis induced by dextran sulphate in germ-free and conventionally reared immunocompetent and immunodeficient mice. Folia Microbiologica. 2001;46:565-572.
42. Maruvada P, Leone V, Kaplan LM, Chang EB. The human microbiome and obesity: Moving beyond associations. Cell Host Microbe. 2017;22(5):589-599.
43. Paun A, Yau C, Danska JS. The influence of the microbiome on type 1 diabetes. J Immunol. 2017;198(2):590–595.
44. Perry RJ, Peng L, Barry NA, et al. Acetate mediates a microbiome-brain-beta-cell axis to promote metabolic syndrome. Nature. 2016;534(7606):213-217.
45. Zhong D, Wu C, Zeng X, Wang Q. The role of gut microbiota in the pathogenesis of rheumatic diseases. Clin Rheumatol. 2018;37(1):25-34.
46. Cryan JF, Dinan TG. Mind-altering microorganisms: The impact of the gut microbiota on brain and behaviour. Nat Rev Neurosci. 2012;13(10):701-712.
47. Fung TC, Olson CA, Hsiao EY. Interactions between the microbiota, immune and nervous systems in health and disease. Nat Neurosci. 2017;20(2):145-155.
48. Cryan JF, O’Riordan KJ, Sandhu K, Peterson V, Dinan TG. The gut microbiome in neurological disorders. Lancet Neurol. 2020;19(2):179-194.
49. Wen L, Ley RE, Volchkov PY, et al. Innate immunity and intestinal microbiota in the development of Type 1 diabetes. Nature. 2008;455(7216):1109-1113.
50. David LA, Maurice CF, Carmody RN, et al. Diet rapidly and reproducibly alters the human gut microbiome. Nature. 2014;505(7484):559- 563.
51. Reid G. Probiotics: Definition, scope, and mechanisms of action. Best Pract Res Clin Gastroenterol. 2016;30(1):17-25.
52. Dusek O, Fajstova A, Klimova A, et al. Severity of Experimental Autoimmune Uveitis Is Reduced by Pretreatment with Live Probiotic Escherichia coli Nissle 1917. Cells. 2020;10(1):23.
53. Kokesova A, Frolova L, Kverka M, et al. Oral administration of probiotic bacteria (E. coli Nissle, E. coli O83, Lactobacillus casei) influences the severity of dextran sodium sulfate-induced colitis in BALB/c mice. Folia Microbiol. 2006;5:478-484.
54. Petschow B, Dore J, Hibberd P, et al. Probiotics, prebiotics, and the host microbiome: the science of translation. Ann NY Acad Sci. 2013;1306:1-17.
55. Jernberg C, Lofmark S, Edlund C, Jansson JK. Long-term ecological impacts of antibiotic administration on the human intestinal microbiota. ISME J. 2007;1:56-66.
56. Nobel YR, Cox LM, Kirigin FF, et al. Metabolic and metagenomic outcomes from early-life pulsed antibiotic treatment. Nat Commun. 2015;6:7486.
57. Cox LM, Yamanishi S, Sohn J, et al. Altering the intestinal microbiota during a critical developmental window has lasting metabolic consequences. Cell. 2014;158:705-721.
58. van Nood E, Vrieze A, Nieuwdorp M, et al. Duodenal infusion of donor feces for recurrent Clostridium difficile. N Engl J Med. 2013;368(5):407-415.
59. Cammarota G, Ianiro G, Tilg H, et al. European consensus conference on faecal microbiota transplantation in clinical practice. Gut. 2017;66(4):569-580.
60. Blank M, Barzilai O, Shoenfeld Y. Molecular mimicry and auto-immunity. Clin Rev Allergy Immunol. 2007;32:111-118.
61. Caspi RR, Roberge FG, Chan CC. A new model of autoimmune disease. Experimental autoimmune uveoretinitis induced in mice with two different retinal antigens, J Immunol. 1988;140:1490- 1495.
62. Luger D, Silver PB, Tang J, et al. Either a Th17 or a Th1 effector response can drive autoimmunity: conditions of disease induction affect dominant effector category. J Exp Med. 2008;205:799-810.
63. Caspi RR, Horai R, Zárate-Bladés CR. Activation of autoreactive T cells by endogenous commensal mikroflora provokes spontaneous autoimmunity in the immunologically privileged eye. Investigat Ophthal Vis Sci. 2014;55:4600-4600.
64. Horai R, Zárate-Bladés CR, Dillenburg-Pilla P, et al. Microbiota-dependent activation of an autoreactive T cell receptor provokes autoimmunity in an immunologically privileged site. Immunity. 2015;43:343-353.
65. Heissigerova J, Seidler Stangova P, Klimova A, et al. The microbiota determines susceptibility to experimental autoimmune uveoretinitis. J Immunol Res. 2016;2016:5065703.
66. Seidler Stangova P, Dusek O, Klimova, Heissigerova J, Kucera T, Svozilkova P. Metronidazole attenuates the intensity of inflammation in experimental autoimmune uveitis. Folia Biol. 2019;65:265-274.
67. Nakamura YK, Metea C, Karstens L, et al. Gut microbial alterations associated with protection. Invest Ophthalmol Vis Sci. 2016;57(8):3747-3758.
68. Kugadas A, Wright Q, Geddes-McAlister J, Gadjeva M. Role of microbiota in strengthening ocular mucosal barrier function through secretory IgA. Investig Ophthalmol Vis Sci. 2017;58:4593- 4600.
69. Nakamura YK, Janowitz C, Metea C, et al. Short chain fatty acids ameliorate immune-mediated uveitis partially by altering migration of lymphocytes from the intestine. Sci Rep. 2017;7:11745.
70. Chen X, Su W, Wan T, et al. Sodium butyrate regulates Th17/Treg cell balance to ameliorate uveitis via the Nrf2/HO-1 pathway. Biochem Pharmacol. 2017;142:111-119.
71. Kim J, Choi SH, Kim YJ, et al. Clinical effect of IRT-5 probiotics on immune modulation of autoimmunity or alloimmunity in the eye. Nutrients. 2017;9(11):1166.
72. Shimizu J, Kubota T, Takada E, et al. Bifidobacteria abundance-featured gut microbiota compositional change in patients with Behcet’s disease. PLoS One. 2016;11: e0153746.
73. Huang X, Ye Z, Cao Q, et al. Gut microbiota composition and fecal metabolic phenotype in patients with acute anterior uveitis. Invest Ophthalmol Vis Sci. 2018;59(3):1523-1531.
74. Kalyana Chakravarthy S, Jayasudha R, Sai Prashanthi G, et al. Dysbiosis in the gut bacterial microbiome of patients with uveitis, an inflammatory disease of the eye. Indian J Microbiol. 2018;58(4):457- 469.
75. Jayasudha R, Kalyana Chakravarthy S, Sai Prashanthi G, et al. Implicating dysbiosis of the gut fungal microbiome in uveitis, an inflammatory disease of the eye. Invest Ophthalmol Vis Sci. 2019;60(5):1384-1393.
76. Ye Z, Wu C, Zhang N, et al. Altered gut microbiome composition in patients with Vogt-Koyanagi-Harada disease. Gut Microbes. 2020;11(3):539-555.
77. Ye Z, Zhang N, Wu C, et al. A metagenomic study of the gut microbiome in Behcet’s disease. Microbiome 2018;6(1):135.
Štítky
OftalmológiaČlánok vyšiel v časopise
Česká a slovenská oftalmologie
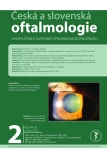
2022 Číslo 2
- Cyklosporin A v léčbě suchého oka − systematický přehled a metaanalýza
- Pomocné látky v roztoku latanoprostu bez konzervačních látek vyvolávají zánětlivou odpověď a cytotoxicitu u imortalizovaných lidských HCE-2 epitelových buněk rohovky
- Konzervační látka polyquaternium-1 zvyšuje cytotoxicitu a zánět spojený s NF-kappaB u epitelových buněk lidské rohovky
- Dlouhodobé výsledky lokální léčby cyklosporinem A u těžkého syndromu suchého oka s 10letou dobou sledování
- Syndrom suchého oka
Najčítanejšie v tomto čísle
- INCIDENCE OF PSEUDOEXFOLIATION SYNDROME AND GLAUCOMA IN A SET OF MORE THAN 14,000 EYES OF PATIENTS OPERATED FOR A CATARACT
- CONTEMPORARY PRACTICES IN CATARACT SURGERY – SUBJECTIVE PREFERENCES OF CZECH CATARACT SURGEONS
- IDIOPATHIC SCLEROCHOROIDAL CALCIFICATIONS. A CASE REPORT
- MICROBIOME AND UVEITIDES. A REVIEW