Effect of DNA Methylation on the Development of Cancer
Authors:
Holčáková Jitka
Authors place of work:
Regionální centrum aplikované molekulární onkologie, Masarykův onkologický ústav, Brno
Published in the journal:
Klin Onkol 2018; 31(Supplementum 2): 41-45
Category:
Přehled
doi:
https://doi.org/10.14735/amko20182S41
Summary
Background:
Research in the last decade has confirmed the importance of epigenetic processes for the onset, development, and treatment of cancer. Next generation sequencing has allowed the inspection and mapping of the human epigenome and its monitoring for changes during carcinogenesis, which has revealed direct links between epigenetic abnormalities and mutations in genes that control DNA methylation and packing and those that function in chromatin dynamics and metabolism. Epigenetic changes that occur in the early stages of tumor progression thus represent promising candidates for diagnostic and prognostic markers, and epigenetic processes are suitable targets for the development of new therapeutic strategies. There are two contrasting views on how aberrant DNA methylation contributes to the development of cancer. The first view assumes that normal cells undergo transformation due to driver mutations and subsequent de novo methylation and DNA demethylation, resulting in global changes in gene expression. The second view considers changes in DNA methylation to be a consequence of cell aging, for example, and that the acquired changes increase the sensitivity of DNA to mutations and oncogenic transformation.
Aims:
The aim of the review article is to briefly summarize the role of abnormal DNA methylation in the development of cancer, and to present an alternative theory that considers the role of aberrant DNA methylation patterns in cancer from a new and unconventional perspective.
Key words:
DNA methylation – polycomb-group proteins – CpG islands
The work was supported by the project MEYS – NPS I – LO1413.
The author declares she has no potential conflicts of interest concerning drugs, products, or services used in the study.
The Editorial Board declares that the manuscript met the ICMJE recommendation for biomedical papers.
Accepted: 27. 8. 2018
Zdroje
1. Jones PA, Baylin SB. The fundamental role of epigenetic events in cancer. Nat Rev Genet 2002; 3 (6): 415–428. doi: 10.1038/nrg816.
2. Kim JK, Samaranayake M, Pradhan S. Epigenetic mechanisms in mammals. Cell Mol Life Sci 2009; 66 (4): 596–612. doi: 10.1007/s00018-008-8432-4.
3. Bird A. DNA methylation patterns and epigenetic memory. Genes Dev 2002; 16 (1): 6–21. doi: 10.1101/gad. 947102.
4. Chen ZX, Riggs AD. DNA methylation and demethylation in mammals. J Biol Chem 2011; 286 (21): 18347–18353. doi: 10.1074/jbc.R110.205286.
5. Poh WJ, Wee CP, Gao Z. DNA Methyltransferase activity assays: advances and challenges. Theranostics 2016; 6 (3): 369–391. doi: 10.7150/thno.13438.
6. Cheng X, Blumenthal RM. Mammalian DNA methyltransferases: a structural perspective. Structure 2008; 16 (3): 341–350. doi: 10.1016/j.str.2008.01.004.
7. Buck-Koehntop BA, Defossez PA. On how mammalian transcription factors recognize methylated DNA. Epigenetics 2013; 8 (2): 131–137. doi: 10.4161/epi.23632.
8. Berman BP, Weisenberger DJ, Aman JF et al. Regions of focal DNA hypermethylation and long-range hypomethylation in colorectal cancer coincide with nuclear lamina-associated domains. Nat Genet 2011; 44 (1): 40–46. doi: 10.1038/ng.969.
9. Bjornsson HT, Fallin MD, Feinberg AP. An integrated epigenetic and genetic approach to common human disease. Trends Genet 2004; 20 (8): 350–358. doi: 10.1016/j.tig.2004.06.009.
10. Issa JP. Epigenetic variation and cellular Darwinism. Nat Genet 2011; 43 (8): 724–726. doi: 10.1038/ng.897.
11. Hansen KD, Timp W, Bravo HC et al. Increased methylation variation in epigenetic domains across cancer types. Nat Genet 2011; 43 (8): 768–775. doi: 10.1038/ng.865.
12. Feinberg AP, Gehrke CW, Kuo KC et al. Reduced genomic 5-methylcytosine content in human colonic neoplasia. Cancer Res 1988; 48 (5): 1159–1161.
13. Chan MF, van Amerongen R, Nijjar T et al. Reduced rates of gene loss, gene silencing, and gene mutation in Dnmt1-deficient embryonic stem cells. Mol Cell Biol 2001; 21 (22): 7587–7600. doi: 10.1128/MCB.21.22.7587-7600.2001.
14. Szyf M, Pakneshan P, Rabbani SA. DNA methylation and breast cancer. Biochem Pharmacol 2004; 68 (6): 1187–1197. doi: 10.1016/j.bcp.2004.04.030.
15. Bestor TH. The DNA methyltransferases of mammals. Hum Mol Genet 2000; 9 (16): 2395–2402.
16. Baylin SB, Herman JG. DNA hypermethylation in tumorigenesis: epigenetics joins genetics. Trends Genet 2000; 16 (4): 168–174.
17. Jones PA, Laird PW. Cancer epigenetics comes of age. Nat Genet 1999; 21 (2): 163–167. doi: 10.1038/5947.
18. Esteller M, Silva JM, Dominguez G et al. Promoter hypermethylation and BRCA1 inactivation in sporadic breast and ovarian tumors. J Natl Cancer Inst 2000; 92 (7): 564–569.
19. Esteller M, Avizienyte E, Corn PG et al. Epigenetic inactivation of LKB1 in primary tumors associated with the Peutz-Jeghers syndrome. Oncogene 2000; 19 (1): 164–168. doi: 10.1038/sj.onc.1203227.
20. Myohanen SK, Baylin SB, Herman JG. Hypermethylation can selectively silence individual p16ink4A alleles in neoplasia. Cancer Res 1998; 58 (4): 591–593.
21. Esteller M, Fraga MF, Guo M et al. DNA methylation patterns in hereditary human cancers mimic sporadic tumorigenesis. Hum Mol Genet 2001; 10 (26): 3001–3007.
22. Jones PA, Baylin SB. The epigenomics of cancer. Cell 2007; 128 (4): 683–692. doi: 10.1016/j.cell.2007.01.029.
23. Zhou C, Li J, Li Q. CDKN2A methylation in esophageal cancer: a meta-analysis. Oncotarget 2017; 8 (30): 50071–50083. doi: 10.18632/oncotarget.18412.
24. Robaina MC, Faccion RS, Arruda VO et al. Quantitative analysis of CDKN2A methylation, mRNA, and p16 (INK4a) protein expression in children and adolescents with Burkitt lymphoma: biological and clinical implications. Leuk Res 2015; 39 (2): 248–256. doi: 10.1016/j.leukres.2014.11.023.
25. Jonsson A, Tuominen R, Grafstrom E et al. High frequency of p16 (INK4A) promoter methylation in NRAS-mutated cutaneous melanoma. J Invest Dermatol 2010; 130 (12): 2809–2817. doi: 10.1038/jid.2010.216.
26. Esteller M, Hamilton SR, Burger PC et al. Inactivation of the DNA repair gene O6-methylguanine-DNA methyltransferase by promoter hypermethylation is a common event in primary human neoplasia. Cancer Res 1999; 59 (4): 793–797.
27. Herman JG, Civin CI, Issa JP et al. Distinct patterns of inactivation of p15INK4B and p16INK4A characterize the major types of hematological malignancies. Cancer Res 1997; 57 (5): 837–841.
28. Wood LD, Parsons DW, Jones S et al. The genomic landscapes of human breast and colorectal cancers. Science 2007; 318 (5853): 1108–1113. doi: 10.1126/science.1145720.
29. Akiyama Y, Watkins N, Suzuki H et al. GATA-4 and GATA-5 transcription factor genes and potential downstream antitumor target genes are epigenetically silenced in colorectal and gastric cancer. Mol Cell Biol 2003; 23 (23): 8429–8439.
30. Suzuki H, Toyota M, Carraway H et al. Frequent epigenetic inactivation of Wnt antagonist genes in breast cancer. Br J Cancer 2008; 98 (6): 1147–1156. doi: 10.1038/sj.bjc.6604259.
31. Nakagawa H, Nuovo GJ, Zervos EE et al. Age-related hypermethylation of the 5‘ region of MLH1 in normal colonic mucosa is associated with microsatellite-unstable colorectal cancer development. Cancer Res 2001; 61 (19): 6991–6995.
32. Esteller M, Risques RA, Toyota M et al. Promoter hypermethylation of the DNA repair gene O (6) -methylguanine-DNA methyltransferase is associated with the presence of G: C to A: T transition mutations in p53 in human colorectal tumorigenesis. Cancer Res 2001; 61 (12): 4689–4692.
33. Coulondre C, Miller JH, Farabaugh PJ et al. Molecular basis of base substitution hotspots in Escherichia coli. Nature 1978; 274 (5673): 775–780.
34. Rideout WM3rd, Coetzee GA, Olumi AF et al. 5-Methylcytosine as an endogenous mutagen in the human LDL receptor and p53 genes. Science 1990; 249 (4974): 1288–1290.
35. Pfeifer GP, Tang M, Denissenko MF. Mutation hotspots and DNA methylation. Curr Top Microbiol Immunol 2000; 249: 1–19.
36. Yoon JH, Smith LE, Feng Z et al. Methylated CpG dinucleotides are the preferential targets for G-to-T transversion mutations induced by benzo[a]pyrene diol epoxide in mammalian cells: similarities with the p53 mutation spectrum in smoking-associated lung cancers. Cancer Res 2001; 61 (19): 7110–7117.
37. Fang M, Ou J, Hutchinson L et al. The BRAF oncoprotein functions through the transcriptional repressor MAFG to mediate the CpG island methylator phenotype. Mol Cell 2014; 55 (6): 904–915. doi: 10.1016/j.molcel.2014.08.010.
38. Issa JP. Aging and epigenetic drift: a vicious cycle. J Clin Invest 2014; 124 (1): 24–29. doi: 10.1172/JCI69735.
39. Raddatz G, Hagemann S, Aran D et al. Aging is associated with highly defined epigenetic changes in the human epidermis. Epigenetics Chromatin 2013; 6 (1): 36. doi: 10.1186/1756-8935-6-36.
40. Keshet I, Schlesinger Y, Farkash S et al. Evidence for an instructive mechanism of de novo methylation in cancer cells. Nat Genet 2006; 38 (2): 149–153. doi: 10.1038/ng1719.
41. Bernstein BE, Mikkelsen TS, Xie X et al. A bivalent chromatin structure marks key developmental genes in embryonic stem cells. Cell 2006; 125 (2): 315–326. doi: 10.1016/j.cell.2006.02.041.
42. Mikkelsen TS, Ku M, Jaffe DB et al. Genome-wide maps of chromatin state in pluripotent and lineage-committed cells. Nature 2007; 448 (7153): 553–560. doi: 10.1038/nature06008.
43. Boyer LA, Plath K, Zeitlinger J et al. Polycomb complexes repress developmental regulators in murine embryonic stem cells. Nature 2006; 441 (7091): 349–353. doi: 10.1038/nature04733.
44. Ohm JE, Baylin SB. Stem cell chromatin patterns: an instructive mechanism for DNA hypermethylation? Cell Cycle 2007; 6 (9): 1040–1043. doi: 10.4161/cc.6.9.4210.
45. Cedar H, Bergman Y. Linking DNA methylation and histone modification: patterns and paradigms. Nat Rev Genet 2009; 10 (5): 295–304. doi: 10.1038/nrg2540.
46. Easwaran H, Johnstone SE, Van Neste L et al. A DNA hypermethylation module for the stem/progenitor cell signature of cancer. Genome Res 2012; 22 (5): 837–849. doi: 10.1101/gr.131169.111.
47. Nejman D, Straussman R, Steinfeld I et al. Molecular rules governing de novo methylation in cancer. Cancer Res 2014; 74 (5): 1475–1483. doi: 10.1158/0008-5472.CAN-13-3042.
48. Tomasetti C, Vogelstein B. Cancer etiology. Variation in cancer risk among tissues can be explained by the number of stem cell divisions. Science 2015; 347 (6217): 78–81. doi: 10.1126/science.1260825.
49. Easwaran H, Tsai HC, Baylin SB. Cancer epigenetics: tumor heterogeneity, plasticity of stem-like states, and drug resistance. Mol Cell 2014; 54 (5): 716–727. doi: 10.1016/j.molcel.2014.05.015.
50. O‘Hagan HM, Wang W, Sen S et al. Oxidative damage targets complexes containing DNA methyltransferases, SIRT1, and polycomb members to promoter CpG Islands. Cancer Cell 2011; 20 (5): 606–619. doi: 10.1016/j.ccr.2011.09.012.
51. Klutstein M, Nejman D, Greenfield R et al. DNA Methylation in Cancer and Aging. Cancer Res 2016; 76 (12): 3446–3450. doi: 10.1158/0008-5472.CAN-15-3278.
Štítky
Detská onkológia Chirurgia všeobecná OnkológiaČlánok vyšiel v časopise
Klinická onkologie
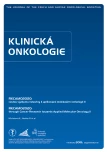
2018 Číslo Supplementum 2
- Metamizol jako analgetikum první volby: kdy, pro koho, jak a proč?
- Nejasný stín na plicích – kazuistika
- Fixní kombinace paracetamol/kodein nabízí synergické analgetické účinky
- Antidepresivní efekt kombinovaného analgetika tramadolu s paracetamolem
- Kombinace metamizol/paracetamol v léčbě pooperační bolesti u zákroků v rámci jednodenní chirurgie
Najčítanejšie v tomto čísle
- Vliv metylace DNA na vznik nádorových onemocnění
- Ferroptóza jako nový typ buněčné smrti a její role v léčbě rakoviny
- Možnosti využití p63 v bioptické diagnostice
- Současné metody analýzy mikroRNA