Effects of vitamin D3, magnesium and vitamin C on bone-associated miRNAs expression in adipose-derived mesenchymal stem cells
Účinky vitamínu D3, horčíka a vitamínu C na expresiu osteo-asociovaných miRNA mezenchýmových kmeňových buniek tukového tkaniva
Úvod: Vitamín D3, horčík, ale aj vitamín C zohrávajú limitovanú rolu vo vápnikovej homeostáze a fyziológii kostí. Okrem iného majú aj pleiotropné účinky na rôzne typy buniek, akými sú napr. mezenchýmové kmeňové bunky odvodené z tukového tkaniva (Adipose-Derived Mesenchymal Stem Cells – ADMSCs).
Cieľ štúdie: Cieľom tejto štúdie bolo získať nový pohľad na vplyv magnézia, cholekalciferolu (vitamínu D3) a kyseliny askorbovej (vitamínu C) na potenciálne zmeny v kultivácii a diferenciácii ADMSCs. Nedávny výskum odhalil zapojenie mnohých mikroRNA (miRNA) pochádzajúcich z kostnej hmoty do regulácie fyziológie/patológie kostí. Preto sme sa tiež zamerali na analýzu zmien expresie miRNA v tomto kultivačnom stave.
Metódy: Jednotlivé vzorky ADMSCs sa kultivovali 24 hodín v kompletnom kultivačnom médiu s prídavkom rôznych koncentrácií vitamínu D3, horčíka a vitamínu C. Zmeny v expresii vybraných miRNA kostnej hmoty (miRNA-1, miRNA-29b, miRNA-133a a miRNA-499) sa určili pomocou reverznej transkripcie polymerázovej reťazovej reakcie (Reverse Transcription Polymerase Chain Reaction – RT-PCR).
Výsledky: Po farmakologickom ovplyvnení buniek pridaním vitamínu D3 sa významne zvýšila expresia všetkých pozorovaných miRNA. Pod vplyvom horčíka bolo zaznamenané štatisticky významné zvýšenie expresie miRNA-1 a miRNA-29b v porovnaní s kontrolnou vzorkou. Zvýšené koncentrácie vitamínu C významne ovplyvnili štruktúru a morfológiu sledovaných buniek, pričom došlo k významným zmenám v expresii miRNA-29b a miRNA-499.
Záver: Táto štúdia potvrdila možnosť pozitívneho farmakologického ovplyvnenia kmeňových buniek vitamínom D3, horčíkom, a taktiež vitamínom C v rámci regulácie expresie miRNA pochádzajúcich z kostnej hmoty.
Klíčová slova:
cholekalciferol – mezenchýmové kmeňové bunky odvodené z tukového tkaniva – miRNA – vitamin C – vitamín D3
Authors:
Sabová Nikola 1; Samáková Adriana 1; Pinček Martin 1; Sprušanský Ondrej 1; Gažová Andrea 2; Kyselovič Ján 3
Authors place of work:
Department of Pharmacology and Toxicology, Pharmacy Faculty, Comenius University in Bratislava, Slovakia
1; Institute of Pharmacology and Clinical Pharmacology, Medical Faculty, Comenius University in Bratislava, Slovakia
2; 5th Department of Internal Medicine, Clinical Research Unit, Medical Faculty, Comenius University in Bratislava and University Hospital Bratislava - Hospital Ruzinov, Slovakia
3
Published in the journal:
Clinical Osteology 2019; 24(1): 27-35
Category:
Summary
Introduction: Vitamin D3, magnesium and also vitamin C have a limited role in calcium homeostasis and bone physiology. Among other things, they have pleiotropic effects on various cell types, such as e.g. adipose-derived mesenchymal stem cells (ADMSCs).
Aim of the study: The goal of the present study was to gain new insight into the impact of magnesium, cholecalciferol (vitamin D3) and ascorbic acid (vitamin C) on potential changes in the cultivation and differentiation of ADMSCs. Recent research has revealed the involvement of number of bone-derived miRNAs in the regulation of bone physiology/pathology. Therefore, we also focused on analysing changes of miRNAs expression in this cultivation condition.
Methods: The individual samples of ADMSCs were cultivated for 24 h in complete culture medium with the addition of various concentrations of vitamin D3, magnesium, and vitamin C. Changes in the expression of selected bone-associated miRNAs (miRNA-1, miRNA-29b, miRNA-133a, and miRNA-499) were determined using reverse transcription polymerase chain reaction (RT-PCR).
Results: After the pharmacological influence of cells with vitamin D3, the expression of all the miRNAs observed was significantly increased. Under the influence of magnesium, a statistically significant increase in miRNA-1 and miRNA-29b expression was recorded comparing to the control sample. Increased concentrations of vitamin C significantly affected the structure and morphology of the monitored cells and there were significant changes in the expression of miRNA-29b and miRNA-499.
Conclusion: This study has confirmed the possibility of positive pharmacological treatment of stem cells with vitamin D3, magnesium and also vitamin C in regulating expression of bone-associate miRNAs.
Keywords:
Vitamin C – cholecalciferol – magnesium – miRNA – vitamin D3
Introduction
Mesenchymal stem cells (MSCs) are adult stem cells traditionally found in the bone marrow. However, MSCs can also be isolated from other tissues, such as placenta, umbilical cord blood, adipose tissue, adult muscle, corneal stroma and dental pulp of deciduous baby teeth [1,2]. Adipose tissue is one of the richest sources of MSCs. There are more than 500 times more stem cells (ADMSCs) in 1 gram of fat than in 1 gram of aspirated bone marrow. Mesenchymal stem cells are multipotent cells that adhere to plastic, have a fibroblast-like morphology, express a specific set of surface antigens and differentiate into adipocytes, chondrocytes, osteoblasts and myocytes [3]. There are a few mechanisms how ADMSCs can repair or regenerate tissues. ADMSCs applied to injured or diseased tissue can excrete cytokines and growth factors that stimulate recovery in a paracrine way [4]. The literature data demonstrate an inverse relationship between the differentiation of adipocyte and osteogenic cells in the culture system and are consistent with the possibility that the regulation of adipogenesis and osteogenesis can occur at the level of a common precursor in vivo [5,6].
Stem cells and miRNA
Microribonucleic acid (microRNA, miRNA) is a recently discovered group of eukaryotic, endogenous, non-coding ribonucleic acid (RNA) that plays a key role in regulating gene expression [7]. The new studies suggest that the exchange of miRNA between cells is an integral part of the communication between MSCs and damaged tissue cells. The transport of miRNA is ensured through the microvesicles (MVs) which are released from the original cell. Thus, the biological fluids can reach the target cell. This exchange of genetic information can lead to a reprogramming of the MSCs phenotype, thereby obtain the MSCs acquire the characteristics of damaged tissue cells. At the same time, MVs from MSCs can also activated regenerative mechanisms in many different types of damaged tissue cells [8].
MiRNAs have been under investigation for the past twenty years and there is lots of information on miRNAs in diseases such as cancer and immunology. Only recently, miRNAs have shown a promise as a mechanism for intervention with respect to diseases of the bone and adipose tissue. MiRNAs regulate bone metabolism and balance of bone homeostasis and therefore in pathology miRNA signaling pathways contribute to the onset and progression of skeletal disorders. In MSCs differentiation, alterations in miRNA expression patterns can differentially promote an osteogenic, adipogenic, or myogenic phenotype [6]. This pivotal experimental work is focused on the examination of miRNA associated with bone and adipose tissue biology and disease.
There is a growing evidence that bone-associated miRNAs (miRNA-1, miRNA-29b, miRNA-133a and miRNA-499) should be modulators of osteoblast-mediated bone formation and osteoclast related bone resorption, thereby contributing to the maintenance of bone mass [9–11]. These miRNAs are only partially able to affect this process. However, by adding additional specific factors and molecules that can drive cell transdifferentiation, this process can be improved and made more effective.
Several experimental studies have shown the ability of vitamin D3 [12], magnesium [13] and vitamin C [14] to improve the differentiation of stem cells. The aim of this study is to evaluate the influence of vitamin D3, magnesium and vitamin C on the morphology of isolated adipose derivated mesenchymal stem cells from adipose tissue and on expression of bone-associate miRNAs (miRNA-1, miRNA-133a, miRNA-29b, miRNA-499).
Material and methods
Isolation and culture of ADMSCs
A sample of human subcutaneous adipose tissue was collected by aseptic liposuction into the PBS + 5% Penicillin/Streptomycin solution (Sigma-Aldrich). Within 24 h the tissue was washed with PBS and after separation of components, it was cut with a sterile scalpel. The fatty tissue was incubated with an equivalent amount of 0.2% collagenase type I (Sigma-Aldrich) for 2 h at 37 °C. Cell pellet and supernatant (oil, fat and adipocytes) were formed by centrifugation at 400 g for 30 min. After the aspiration of the top layers the pellet was washed in PBS + 0.1% BSA (Sigma-Aldrich) and resuspended in 10 ml complete culture medium (CCM) consisting of DMEM/F12 + 10% foetal bovine serum + 5% Penicillin/Streptomycin (all from Sigma-Aldrich). The cells were then cultured in a 75 cm2 flasks at 37 °C with 5% CO2 /95% air atmosphere. Non-adherent hematopoietic cells were removed by medium change every 24 h for 3 days. The complete culture medium was replaced every 2 days until the cells reached 80–90% confluence. Afterward, the cultures were passaged.
Flow cytometry analysis
Quantitative and qualitative characterization of the cells was made utilizing a flow cytometry method (MACSQuant Analyzer, Miltenyi Biotec, Germany). The MSC Phenotyping Kit/human, isotype control cocktail and CD34/CD133 Stem Cell Cocktail with control cocktail (all from Miltenyi Biotec) were used to detect and quantify ADMSCs. In human MSC Phenotyping cocktail the monoclonal antibodies are conjugated to the following fluorochromes: CD90-FITC; CD105 – PE; CD73 – APC; CD14, CD20, CD34, CD45 – PerCP. At the same time the presence of surface markers CD34 and CD133 were examined by CD34 / CD133 Stem Cell Coctail. The MSC Phenotyping kit allows identification of MSC cells by positive staining for markers CD90, CD 105 and CD73. The intercalating agent Propidium iodide -PI (Miltenyi Biotec) was used to determine the viability of the cells. PI binds to nucleic acids after passing through broken membranes of dead cells and this binding is detectable by suitable fluorescence detectors.
Individual cell cultures of ADMSCs were released from the bottom of the culture flask by 0.25% trypsin. Subsequently, they were diluted with CCM to a volume of 10 ml, transferred to a falcon (15 ml) and centrifuged at 300G for 10 minutes. After removing the supernatant, the pellet was resuspended in 1 ml of PBS and centrifuged (300G, 10 minutes). Washing with PBS was repeated three times and pellet was finally resuspended in 400 μl AutoMACS Running Buffer. 50 μl of a well-diluted sample (about 1 × 106–107 cells/ml) was incubated with 5 μl of antibody for 10 minutes at 4 °C. Subsequently, 450 μl of the AutoMACS Running Buffer was added and just before measuring withal 5 μl of PI in each sample. For the identification of the population manual “gating” strategy on two-dimensional spot points was used.
Culturing with selected drugs
For the experiment, the sixteenth passage (16p) of ADMSCs was used. These cells were cultured for 24 h in CCM supplemented with vitamin C (Acidum ascorbicum sol. inj., Biotika), magnesium (Magnesium sulfuricum, sol.inj., Biotika) and vitamin D3 (Vigantol gtt., Merck). Drugs were added at different concentrations calculated based on the declared therapeutic doses commonly used in clinical practice. Based on this, the analysed samples were divided in the table. To record any structural and morphological changes, individual samples were observed under an inverse microscope.
Real-time RT-PCR
The reverse transcription method followed by polymerase chain reaction (RT-PCR) is used when the starting material is RNA. In this case, transcription from total RNA or mRNA to complementary DNA (cDNA) must be performed by the reverse transcriptase enzyme. The cDNA then serves as template for the PCR reaction. After 24 h incubation with selected drugs the total RNA was extracted from 10.000 of ADMSC-cells using TRI Reagent™ Solution (Invitrogen™) according to the manufacturer’s instructions and using of phenol-chloroform extraction. For quantitative real-time reverse-transcription PCR analysis, a TaqMan MicroRNA Reverse Transcription Kit (Applied Biosystems) was used. Quantitative PCR was performed with the TaqMan Universal PCR Master Mix II (Applied Biosystems). Each sample was analysed in triplicate. Every batch of amplifications included 3 water blanks as negative controls for each of the reverse-transcription and PCR steps. All data were collected and analysed with LinReg PCR. We conducted TaqMan MicroRNA Assays for hsa-miR-1, hsa-miR-29b-3p, hsa-miR-133a-3p, miR-449–5p and U6 snRNA (Invitrogen™). Statistical analyses were performed with T-Test.
Results
Flow cytometry analysis
MSCs isolated from human adipose tissue were characterized by positive staining for markers CD90, CD105 and CD73, and negative staining for markers CD14, CD20, CD34 and CD45 (figure 1). The viability and amounts of cells positive for MSC-markers were determined after selection of “debris”, clusters of cells and dead cells. The viability of cells was more than 90% in all treated cells.
Structure and morphology of the ADMSCs
After 24 h of culture, the structure and morphology of ADMSCs-16p cells were observed under an inverse microscope. Cells in control sample cultured in pure CCM showed no significant changes compared to the previous day. No significant structural and morphological changes were also detected in cells treated with two concentrations of vitamin D3 and magnesium. Cells were similar in appearance to the control sample. However, changes were observed in samples vitamin C 25 mM and 50 mM. The cells changed their appearance and their ability to adhere to the plastic was significantly weakened (figure 2).
The effect of selected drugs on miRNA-1 expression
No significant changes in miRNA-1 expression were recorded in vitamin C 25 mM and 50 mM samples. However, more significant effects on the expression of this miRNA had vitamin D3 and magnesium. In the case of vitamin D3, approximately the same rate of miRNA-1 expression was observed at both used concentrations. Upon cultivation of the cells with magnesium a more pronounced increase in miRNA-1 expression was observed in sample magnesium 40 mM (figure 3).
The effect of selected drugs on miRNA-133a expression
In the case of miRNA-133a, its increased expression was seen against the control sample in all cases. The most pronounced increase in expression was for the sample magnesium 89 mM. A significant increase in the expression was also observed for samples of vitamin D3 at both used concentrations. A gradual increase in expression was recorded for samples cultured with vitamin C. Expression increased directly in proportion to the increasing concentrations with a statistically significant change in sample vitamin C 50 mM (figure 4).
The effect of selected drugs on miRNA-29b expression
As with miRNA-133, the miRNA-29b expression analysis also showed an increase in all pharmacologically-affected samples compared to control sample. Individual drugs at all used concentrations resulted in significant increase in the expression of this miRNA.
The most striking results were observed after culturing the cells with vitamin C. Already at the lowest concentration there was a significant increase in the expression of miRNA-29b. At higher concentrations of vitamin C, expression differences were even more remarkable comparing to the control sample. After the cultivation of the cells with magnesium, there was also a significant increase in miRNA-29b expression at both used concentrations, but a more pronounced increase in expression was observed at a lower concentration of magnesium (40 mM). The effect of two used concentrations of vitamin D3 on miRNA-29b expression was approximately the same (figure 5).
The effect of selected drugs on miRNA-499 expression
The results of miRNA-499 expression in the samples were very heterogeneous comparing to other miRNAs. Under the influence of vitamin C there was a decrease in the expression of this parameter, most notably in the sample vitamin C 25 mM. Conversely, increased expression of miRNA-499 was observed in samples cultured with vitamin D3. Almost the same level of miRNA-499 expression was achieved at both concentrations of this drug. Interesting was the evaluation of changes in expression of this miRNA in cells after 24 hours of cultivation with magnesium. While a significant decrease in miRNA-499 expression was observed in the sample magnesium 40 mM, there was a slight increase in expression in the sample magnesium 89 mM relative to the control sample. However, this change was not statistically significant (figure 6).
Discussion
In our study we worked with the 16th passage of ADMCs, which showed almost 100% uniformity in the positivity characteristic for CD90, CD105 and CD73 markers, and negativity for CD14, CD20, CD34 and CD45 markers, as reported in the methodology. So far there is only one published work [15], which reports the same MSC potential from different types of mesenchymal cell isolation from adipose tissue. Also, in the process of purifying MSC from adipose tissue, all types of adipose tissue cells (both white and brown fat) and all published papers reported only a single type of ADMSCs, characterized by the above-mentioned markers. And so, we do in our study.
Growing experimental data confirm the key role of miRNAs in regulation of bone metabolism and balance of bone homeostasis. MiRNAs play a crucial role in repressing or promoting the expression of specific mRNAs involved in the signaling pathways of cell differentiation and therefore in pathology, miRNA signaling pathways may contribute to the onset and progression of skeletal disorders. In ADMSCs differentiation, alterations in miRNA expression patterns can differentially promote an osteogenic, adipogenic or myogenic phenotype [3] and miRNAs expression can shift the balance between adipogenesis and osteogenesis, e.g. miR-17, miR-204 and miR-211 modulate switching between specific phenotype cell’s [16–18]. Therefore, it is important to search difference in miRNAs expression between differentiate down a specific lineage myogenic, adipogenic or osteogenic to push the balance and favour phenotype [6]. A comprehensive recognition of the biogenesis of miRNAs during differentiation of ADMSCs may give greater insight into mechanisms for intervention and treatment of different diseases. This is the first evidence showing that ADMSCs during in vitro activation significantly changed expression of miRNAs associate with bone pathology (miRNA-1, -29b, -133, and -499) [9–11].
MiRNAs are only partially able to affect the cell´s differentiation process. However, a specific pharmacological intervention should be able to drive cell transdifferentiation, and this process can be improved and made more effective. Several experimental studies have shown the ability of vitamin D3 [12], magnesium [13] and vitamin C [14] to improve the differentiation of stem cells. In our experiment after a short-term (24 hours) incubation of the cells with vitamin C no significant effect on the expression of miRNA-1 was observed. But the levels of miRNA-133a were increased proportionally to the concentration of added vitamin C. Statistically significant increase compared to control sample was recorded in the sample with the highest concentration of vitamin C – 50 mM. MiRNA-1 and miRNA-133a are preferentially expressed in cardiac and skeletal muscle, but there is first evidence that they could take part also in the regulation of differentiation and proliferation of ADMSCs [19]. In our experimental cells, under the influence of vitamin C, changes in miRNA-499 expression were also observed. In this case a negative effect of vitamin C was observed and the expression of this miRNA was statistically significantly reduced comparing to the control sample. The physiological as well as pathological bone function of miRNA-499 was described only recently and is still very questionable [9,20]. Only in the case of miRNA-29b a significant increase in its expression under the influence of vitamin C were observed. Overexpression and delivery of miR-29 family members promote osteoblast differentiation and bone formation, and inhibit osteoclast differentiation [10,21].
Much greater increase in the expression of miRNA-1 and miRNA-133a was observed after the addition of vitamin D3. Vitamin D3 in culture conditions also significantly increased the expression of miRNA-499. Interestingly, both concentrations of used drug (0.05 mM and 0.5 mM) affected the expression of the referred miRNA about the same extent. Similarly, expression of miRNA, was evaluated and recorded in the case of cells which were cultured for 24 h in media with addition of two different magnesium concentrations. A study demonstrated the positive effect of magnesium on growth and proliferation of human stem cells. After addition of various concentrations of magnesium in the form of magnesium salts, increased number of viable cells over time was observed in the samples with higher concentrations of magnesium [22]. In the evaluation of our results, varied effect of two magnesium concentrations (40 mM and 89 mM) was observed for the expression of all the miRNAs. Under the influence of magnesium, the expression of the three miRNAs was increased, namely miRNA-1, miRNA-133a and miRNA-29b. Whereas the expression of miRNA-1 and miRNA-29b was more profound in the experimental samples treated by lower concentration of magnesium, miRNA-133a had more increase in expression with concentration 89 mM.
In conclusion, our study has confirmed the possibility of influencing isolated adipose derivated mesenchymal stem cells not only by altered conditions, but also by pre-treatment of incubated cells with drugs. After the pharmacological intervention of vitamin D3, magnesium and vitamin C the expression of the observed miRNAs (miR-1, -29b, -133, -499) was significantly modified. Because the alterations in miRNA expression patterns are involved in mechanisms where ADMSCs can repair or regenerate tissues, the pre-treatment of stem cells during culturing prior to their transplantation could be a new and effective strategy for the cell therapies.
Acknowledgements
This study was supported by the grants: APVV-14–0416. MZ SR 2016/13-FAUK-1.
PharmDr. Nikola Sabová
Received 15. 5. 2019
Accepted 10. 6. 2019
Zdroje
- Branch MJ, Hashmani K, Dhillon P et al. Mesenchymal stem cells in the human corneal limbal stroma. Invest Ophthalmol Vis Sci 2012; 53(9): 5109–5116. Dostupné z DOI: <http://dx.doi.org/10.1167/iovs.11–8673>.
- Wang S, Qu X, Zhao RC. Clinical applications of mesenchymal stem cells. J Hematol Oncol 2012; 5: 19. Dostupné z DOI: <http://dx.doi.org/10.1186/1756–8722–5-19>.
- Dominici M, Le Blanc K, Mueller I et al. Minimal criteria for defining multipotent mesenchymal stromal cells. The International Society for Cellular Therapy position statement. Cytotherapy 2006; 8(4): 315–317. Dostupné z DOI: <http://dx.doi.org/10.1080/14653240600855905>.
- Gimble JM, Katz AJ, Bunnell BA. Adipose-derived stem cells for regenerative medicine. Circ Res 2007; 100(9): 1249–1260. Dostupné z DOI: <http://dx.doi.org/10.1161/01.RES.0000265074.83288.09>.
- Beresford JN, Bennett JH, Devlin C et al. Evidence for an inverse relationship between the differentiation of adipocytic and osteogenic cells in rat marrow stromal cell cultures. J Cell Sci 1992; 102(Pt 2): 341–351.
- Martin EC, Qureshi AT, Dasa V et al. MicroRNA regulation of stem cell differentiation and diseases of the bone and adipose tissue: Perspectives on miRNA biogenesis and cellular transcriptome. Biochimie 2016; 124: 98–111. Dostupné z DOI: <http://dx.doi.org/10.1016/j.biochi.2015.02.012>.
- Felekkis K, Touvana E, Stefanou Ch et al. MicroRNAs: A newly described class of encoded molecules that play a role in health and disease. Hippokratia 2010; 14(4): 236–240.
- Collino F, Bruno S, Deregibus MC et al. Chapter Fourteen – MicroRNAs and Mesenchymal Stem Cells. Vitam Horm 2011; 87: 291–320. Dostupné z DOI: <http://dx.doi.org/10.1016/B978–0-12–386015–6.00033–0>.
- Liu J, Huang L, Su P et al. MicroRNA-499a-5p inhibits osteosarcoma cell proliferation and differentiation by targeting protein phosphatase 1D through protein kinase B/glycogen synthase kinase 3β signaling. Oncol Lett 2018; 15(4): 4113–4120. Dostupné z DOI: <http://dx.doi.org/10.3892/ol.2018.7814>.
- Taipaleenmäk H. Regulation of Bone Metabolism by microRNAs. Curr Osteoporos Rep 2018; 16(1): 1–12. Dostupné z DOI: <http://dx.doi.org/10.1007/s11914–018–0417–0>.
- Fujii R, Osaka E, Sato K et al. MiR-1 Suppresses Proliferation of Osteosarcoma Cells by Up-regulating p21 via PAX3. Cancer Genomics Proteomics 2019; 16(1): 71–79. Dostupné z DOI: <http://dx.doi.org/10.21873/cgp.20113>.
- Basoli V, Santaniello S, Cruciani S et al. Melatonin and Vitamin D Interfere with the Adipogenic Fate of Adipose-Derived Stem Cells. Int J Mol Sci 2017; 18(5). pii: E981. Dostupné z DOI: <http://dx.doi.org/10.3390/ijms18050981>.
- Sargenti A, Castiglioni S, Olivi E et al. Magnesium Deprivation Potentiates Human Mesenchymal Stem Cell Transcriptional Remodelling. Int J Mol Sci 2018; 19(5). pii: E1410. Dostupné z DOI: <http://dx.doi.org/10.3390/ijms19051410>.
- D’Aniello C, Cermola F, Patriarca EJ et al. Vitamin C in Stem Cell Biology: Impact on Extracellular Matrix Homeostasis and Epigenetics. Stem Cells Int 2017; 2017: 8936156. <http://dx.doi.org/10.1155/2017/8936156>.
- Schneider S, Unger M, Van Griensven M et al. Adipose-derived mesenchymal stem cells from liposuction and resected fat are sources for regenerative medicine. Eur J Med Res 2017; 22(1): 17. Dostupné z DOI: <http://dx.doi.org/10.1186/s40001–017–0258–9>.
- McGregor RA, Choi MS. MicroRNAs in the regulation of adipogenesis and obesity. Curr Mol Med 2011; 11(4): 304–316.
- Alexander R, Lodish H, Sun L. microRNAs in adipogenesis and as therapeutic targets for obesity, Expert Opin Ther Targets 2011; 15(5): 623–636.
- Huang J, Zhao L, Xing L et al. MicroRNA-204 regulates Runx2 protein expression and mesenchymal progenitor cell differentiation. Stem Cells 2010; 28(2): 357–364. Dostupné z DOI: <http://dx.doi.org/10.1002/stem.288>.
- Divakaran V, Mann DL. The emerging role of microRNAs in cardiac remodelling and heart failure. Circ Res 2008; 103(10): 1072–1083. Dostupné z DOI: <http://dx.doi.org/10.1161/CIRCRESAHA.108.183087>.
- Kloosterman WP, Steiner FA, Berezikov E et al. Cloning and expression of new microRNAs from zebrafish. Nucleic Acid Res 2006; 34(9): 2558–2569. Dostupné z DOI: <http://dx.doi.org/10.1093/nar/gkl278>.
- Feichtinger X, Muschitz Ch, Heimel P et al. Bone-related circulating microRNAs miR-29b-3p, miR-550a-3p, and miR-324–3p and their association to bone microstructure and histomorphometry. Sci Rep 2018; 8(1): 4867. Dostupné z DOI: <http://dx.doi.org/10.1038/s41598–018–22844–2>.
- Nguyen TY, Garcia S, Liew CG et al. Effects of magnesium on growth and proliferation of human embryonic stem cells. Conf Proc IEEE Eng Med Biol Soc 2012;2012: 723–726. Dostupné z DOI: <http://dx.doi.org/10.1109/EMBC.2012.6346033>.
Štítky
Clinical biochemistry Paediatric gynaecology Paediatric radiology Paediatric rheumatology Endocrinology Gynaecology and obstetrics Internal medicine Orthopaedics General practitioner for adults Radiodiagnostics Rehabilitation Rheumatology Traumatology OsteologyČlánok vyšiel v časopise
Clinical Osteology
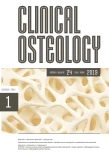
2019 Číslo 1
- Advances in the Treatment of Myasthenia Gravis on the Horizon
- Memantine Eases Daily Life for Patients and Caregivers
- Spasmolytic Effect of Metamizole
- Metamizole at a Glance and in Practice – Effective Non-Opioid Analgesic for All Ages
Najčítanejšie v tomto čísle
- Sarcopenia: definition and diagnosing of a new disease
- Effect of vitamin K on musculoskeletal health in postmenopausal women
- Effect of long-term treatment with growth hormone on bone density and bone quality
- Importance of bone densitometry in cardiovascular risk prediction