Lectins from Eichornia crassipens and Lemna minor may be involved in Vibrio Cholerae El Tor adhesion
Authors:
E. Córdoba-Aguilar 1; R. Coutiño-Rodríguez 1; H. Giles-Ríos 1; P. Hernández-Cruz 2; Mosqueda-Aguilar A(✝); P. Ríos-Cortés 1; H. Montero 1
Authors place of work:
Instituto de Salud Pública, Universidad Veracruzana, Av. Luis Castelazo Ayala s/n. Col. Industrial Ánimas, 91190, Xalapa, Veracruz, México
1; Facultad de Medicina, Universidad de Oaxaca, Oaxaca, México
2
Published in the journal:
Epidemiol. Mikrobiol. Imunol. 67, 2018, č. 1, s. 24-30
Category:
Original Papers
Summary
Vibrio cholerae (Vc) has been isolated from roots of aquatic plants during epidemic or interepidemic periods. It has been suggested that the lectins from the roots of aquatic plants play a role as reservoirs of Vc. In this paper, we evaluated the activity of lectins from Lemna minor and Eichornia crassipens plants as potential mediators of the Vc strain El Tor (Vct). We found that Lemna minor extract showed high specificity towards blood groups O and B. Eichornia crassipens extract showed high specificity towards blood group A and O. Sugar competition experiments demonstrated that Lemna minor extract showed a high recognition to Neu5Ac (acid N acetyl neuraminic or sialic acid) and GlcNAc (N-acetyl D glucoseamine) in group B; and GlcNAc in group O. Eichornia crassipens, the recognition was that of GalNAc (N-acetyl-D-galactoseamine) and GlcNAc in group O; and Fuc (L-Fucose) and GlcNAc in group A.
Lemna minor and Eichornia crassipens protein extracts (p-ext) increased Vct proliferation and protected to the red cells group O against the hemolytic activity of Vct. Both p-exts did not show any statistical significance on agglutination to Vct when compared to the results from phosphate buffer. According to the results, lectins present in roots may be involved in the proliferation and survival of Vct.
Keywords:
Vibrio cholera – Lemna minor – Eichornia crassipens – lectins
INTRODUCTION
Bacteria such as Vibrio cholerae (Vc) are rarely found in the planktonic or free-swimming phase in aquatic environments, they are found in association with a solid surface, such as the exoskeletons of crustaceans or insects, which provide nutritional factors. Vc has been isolated from coastal and estuarine environmental samples, both as free-living and in association with phytoplankton, zooplankton, crustaceans, mollusks and algae [1–5].
Vc is capable to colonize surfaces where are present chitin, which may provide it nutrition directly, because this bacterium is able to metabolize chitin or mucin [6, 7]. Chitin, is one of the most abundant biopolymer of GlcNAc (N-acetyl D glucoseamine) in the aquatic environments, is an important substrate for Vc and has impact on its physiology, including the utilization of carbon and nitrogen source as well as an inductor of natural competence [6, 7].
The capacity of the Vc to respond to changes of salinity, temperature and the availability of nutrients, shows that this species can successfully occupy one or more ecological niches in a variety of aquatic habitats including the human intestine. Vc is the causal agent of severe secretory diarrhea disease named cholera, that means that the life cycle of clinically relevant Vc strains are in transition between these two dissimilar habits: on other words in a natural inhabitant of the aquatic ecosystems and as a pathogen in the human gastrointestinal tract.
The cholera is a reemergence diseases in several countries and in about 50 countries is epidemic [8], and it is transmitted by fecal-oral route thought water. For this reason, it is necessarily to understand its permanency on aquatic niches during interepidemic and the epidemic periods, because nature of cholera latency depends to keep in a viable form, but not necessarily cultivable, in ecological aquatic niches through factors that keep and protected it. A mechanism used by Vc is the formation of biofilms in natural aquatic medium, which favors its survival and transmission, also provide a stable environment and maintains diffusion vibrions large number of microorganisms [9, 10]. In most aquatic and physiologic environment, Vc forms biofilms on surfaces provided by plants, algae, zooplankton, crustaceans and insects, also several gram negative and gram positive pathogens bacteria have the ability to form matrix-enclosed surface associated communities and in particular those biofilms play an important role in the physiology, ecology and epidemiology of cholera [11, 13].
The matrix of the biofilms mainly consists in exopolysaccharides o extracellular polysaccharide (EPS), and other compounds like protein, nucleases (Dns and Xds), lipid and extracellular DNA (eDNA). All this compounds participate in diverse processes including the development of typical biofilm architectures, nutrient acquisition, detachment, dispersion from the biofilms and the colonization fitness of biofilms after ingestion by host; in other words the biofilm enhancing colonization to new niches and facilitating transmission of the disease [11, 12]. There are strains of Vc characterized by being rugose, in that case, the biofilm formation is made in combination with the pilli, the flagello and EPS, also are involved nucleases, lipid, proteins and eDNA [14, 15].
Vc produces a unique EPS, mainly of glucose, galactose, GlcNAc, mannose, xylose and deoxy D galactose, which confers resistance to chloride and promotes biofilm formation. EPS synthesis is controlled by virulence genes (VPS). It has been described two types of Vc strains which respond to different environmental factors: VPS dependent and VPS independent. In VPS dependents strains are necessary factors presents in freshwater; on the contrary, in the independent VPS strain those factors are in salad water [14–16]. The different factors environmental requirements for biofilm formation play different roles in these habitats, which participate in their pathogenicity, life style, survival [7, 10].
Lectins have high specificity for sugar recognition and are implicated in bacterial agglutination, adhesion, pathogenesis and in the biofilm formation by increasing their ability to persist under stress [17–19]. The bacterial fimbriae lectins facilitate cell-cell interactions and are involved in biofilm surface-adhesion. In addition, there are other matrix associated lectin-binding proteins that recognize and bind carbohydrate moieties that favored cell-matrix or cell-cell interactions within the biofilm [10].
The presence of lectins with high specificity for GlcNAc has been described in Vc, able to colonize chitin-containing surfaces [7]. Also it has been described a type of lectin from the serum and mucus of oysters with high specificity to agglutinate the toxigenic Vc [20].
Vc variant El Tor (Vct) has been isolated from the roots of aquatic macrophytes such as Eichornia crassipens and Lemna minor; this suggests that aquatic plants could be natural environmental reservoirs of the microbes through either a non-specific association or a commensal relationship [1–5].
Microbiological monitoring studies in the Actopan River in Veracruz, México demonstrated the presence of Vibrio parahaemolyticus and Vibrio cholerae in roots from Lemna minor and Eichornia crassipens plants [21]. Monitoring the same places after 2 years the results indicated that one of the isolation strains corresponded to Vct [22], their presence may be due to the explosive outbreaks of Vct responsible for the seven pandemics. Several years in the same places Vct is again isolated in a viable and cultivable form from Lemna minor and Eichornia crassipens roots suggesting that these aquatic plants behave as permanent reservoirs of Vct in freshwater [23]. In this work was assayed if the lectins from Lemna minor or Eichornia crassipens roots may be involved in maintaining the association commensal with Vct.
MATERIALS AND METHODS
Plants collection
Plants were collected in sterile bags from Ursulo Galvan river in Veracruz State, roots were separated and rinsed twice with chloride solution to isolate and to identify Vct and also to obtain the p-ext from the roots. In addition, water sample was collected in 500 ml sterile flasks in order to detect free Vct.
Extraction of proteins
To 100 gr of each plant root from Lemna minor and Eichornia crassipens were added 200 ml of petroleum ether in order to remove lipids; this was incubated for 30 min at room temperature. After being delipidized, the sample was macerated in 250 ml of buffer 10 mM phosphate, pH 7 and 125 mM sodium chloride. The maceration obtained was filtered with a gauze; to the filtrate were added several drops of trichloroacetic acid (TCA) to 10% to precipitate proteins, then centrifuged for 15 min at 7000 rpm, each pellet was washed twice with water distilled to remove excess acid, soluble proteins such as albumin in water and soluble carbohydrates. Aliquots of 10 ml phosphate buffer were stored at 0 °C.
The protein concentration of the extracts (p-ext) was quantified by Lowry procedure, utilizing 100 µg/ml of bovine albumin as standard. The sugar concentration was determinated by the Smith method, using 100µg/ml of glucose as standard.
Vc detection
The roots from Lemna minor and Eichornia crassipens were rinsed and incubated directly in alkaline peptone pH 8.5 with 1% saline solution incubate at 45 °C, during 48 hrs to recuperate only Vibrio cholerae, Vct is termoestable. The p-exts were incubated in the same way in alkaline peptone, and them sowing into thiosulphate citrate bile salts (TCBS) medium or directly the roots were collocated on the TCBS, the incubation was about 24 to 72 hrs in order to identify Vc colonies. The Vibrio Cholerae sp was donated and isolated from a patient with Cholerae of the Centro de Especilidades Médicas de Veracruz. The Vct was isolated from the roots of the Lemna minor and Eichornia crassipes and characterized as Inaba [23].
Erythrocytes from groups O, A and B obtaining
Erythrocytes from healthy human donors were collected in heparinized syringes and washed 3 times in PBS (phosphate buffer); finally, a suspension of 5% of red cells was prepared for hemagglutination test.
Hemagglutinating activity (HA)
HA of the extracts was evaluated by modified Coutiño procedure [18], using microplates with 50 μl of 5% red cell suspension from groups A, B and O, in 50 μl of phosphates buffer, followed were added 50 μl of p-ext using the double dilution procedure. HA titer obtained after 1 hour is expressed as the highest dilution showing detectable Hemagglutination. HA units are expressed as the minimum concentration of protein necessary to obtain HA.
Lectin hemagglutinating activity inhibition (HAI) by sugars
The procedure was similar to that for HA, except in this case 1mM of various sugars in double serial dilutions was mixed with a lectin solution at 1:8 titer and incubated for 30 min before the 5% suspension of red cells was added. Ten sugars were tested: GalNAc (N-acetyl-D-galactoseamine), Gal (Galactose), Fuc (L-Fucose), GlcNAc (N-acetyl D glucoseamine), Man (Mannose), Glc (Glucose), Neu5Ac (acid n acetyl neuraminic or sialic acid), Xyl (Xylose), GalN (galactosamine) and GlcN (glucoseamine) (Sigma chemicals, St Louis, Missouri).
SDS-page
Gels at 10% were prepared with or without mercaptoethanol. 50μgr/ml of protein from extract of Lemna minor and Eichornia crassipens roots were added. To determine molecular weight, pre-stained standards (BioRad) and cross-linkage hemoglobin were used (Sigma chemicals), and after stained with coomassie. The resulting bands were analyzed with the Kodak 40 program Digital Sciences 1D.
Inhibition of Vc hemolytic activity by roots p-ext
Vc donate was previously typified [23] and then was cultivated in alkaline peptone water pH 8.5 and inoculated in a nonselective medium such as TSI. After 24 hrs at 37 oC, the bacterial suspension was centrifuged at 3000 rpm for 15 min, 50 μl of the supernatant were used as a source of hemolysin and mixed with 50 μl of 2.5% erythrocyte suspension prepared in an isotonic solution. Vc hemolysin was detect until a titer of 1 : 128. Several double dilutions from Lemna minor and Eichornia crassipens extracts were used to verify hemolytic activity inhibition from the Vc (at titer of 1 : 128), after 30 min of incubation at 37 oC.
Proteic extracts activity on Vct proliferation
SensiDiscs were made with different dilutions of p-ext from Lemna minor and Echornia crassipens, and placed at the corners of the zig-zag proliferation pattern of Vct.
Vc agglutination by roots p-ext
The procedure was similar to that for HA, except that a 2% bacterial suspension of Vct replaced the erythrocytes and saline; polyvalent serum and phosphate buffer were used as positive and negative controls. The Vct aggregation was directly observed by microscope. Determinations also were done in microplates incubated for 30 min, stained with acridine orange and read at 560 nM, corroborated through microscopy analysis. As positive control polyvalent serum and phosphate buffer were used and isotonic solution as negative controls.
RESULTS
Plants obtaining and molecular weight characterization of p-ext
The plants Eichornia crassipes and Lemna minor were collected from Ursulo Galvan river in Veracruz State. Both plants were identified in the Institute of Ecology’s herbarium as was reported [23] and in from their roots p-ext were obtained like is described in materials and methods. The amount of protein and carbohydrates in both extracts were analyzed and it was found that amount of proteins in both extracts was 177 μgr/gr of root, and the carbohydrate concentration was 55 μgr/gr in Eichornia crassipens and 44 μgr/gr in Lemna minor (Table 1). Protein concentration in Lemna minor decreases with time.
To determine the protein pattern of the p-exts, SDS-page was performed. The results in the table 4 show that p-ext from Eichornia crassipens remained the same three bands with and without mercaptoethanol; with molecular weight around 265 kD, 65 kD and 30 kD. Regarding Lemna minor some bands changed its electrophoretic mobility by using mercaptoethanol. Without mercaptoethanol only three bands 191 kD, 66 kD and 16kD were observed, with mercaptoethanol use, four bands: 186 kD, 66 kD, 56 kD and 15.9 kD were observed. The above means that the bands of 191 kD and 66 kD are made up of two polypeptides, one of them about 186 and 56 kD attached to another polypeptide that not detected in the gel probably by its low molecular weight.
Isolation and persistence of Vc
For the Vc isolation, the roots and the p-ext were sowing on TCBS. After two days, yellow and green colonies very homogeneous were observed also few big yellow brownish colonies were observed too. For the identification of strains of Vc, the size and coloration of colony were analyzed [21], based on this classification three strains were detected: Vibrio cholerae, Vibrio parahaemolyticus and Vibrio alginolyticus both the roots and the p-ext (Table 2).
In the water sample after centrifuge at 7,000 rpm in the pellet we didn’t found any colonies, these results corroborate that Vibrio cholerae rarely found in the planktonic or free-swimming phase at least in fresh water and suggest that those plants are permanent reservoirs perhaps they lives in a commensal o symbiotic association [5, 24].
After keep at 4 °C for five years, the p-ext and roots from Lemna minor and Eichornia crassipens, only Vibrio colonies were detected in Eichornia crassipens in both conditions, corroborating their permanency under stress conditions (see Table 2). It must mention that the roots from Lemna minor become dust and in Eichornia crassipens the roots were preserved intact.
It will be mention that when we added antibody poly-O and Inaba to roots to corroborate previous results [23], and incubation for about 5 days Lemna minor, unexpectedly the roots disappears, but before it forms a mucin, probably with the stress of the antibody Vibrio activated a protease activity such as mucinase.
Echornia crassipens and Lemna minor show differents activity of lectins
Lectin activity was determined by the HA test in A, B and O groups. Eichornia crassipens p-ext showed higher activity in group A, with 52,717 units HA/mgr of protein compared to 101 units HA/mgr protein in group O. Lemna minor shows more activity in group O, with 75,294 units HA/mgr and 18,823 units HA/mgr in group B. The results suggest that the p-ext have different lectin activity; and that Eichornia crassipens lectins detected a more specifically a sugar residue in red cells in group A, and Lemna minor detected a different sugar residue in red cells from group O (Table 3).
Sugar specificity of the lectin activity in proteic extracts from plant roots
To know which sugar could inhibit the hemmaglutination between the p-ext and the red cells, ten different sugars were tested: GalNAc, Gal, Fuc, GlcNAc, Man, Glc, Neu5Ac, Xyl, GalN and GlcN. The results from the HAI show that the Eichornia crassipens p-ext, in group A, was inhibited by Fuc and GlcNAc at 1 mM; and in group O, by GlcNAc, GalNAc at 500 µM; and Fuc, Neu5Ac, GlucN, GalN and Gal at 1 mM. Regarding the analysis of HAI by Lemna minor, inhibitors were in group O all sugars tested at 1 mM except GalNac 125 mM; and in group B, the inhibitors were GlcNAc at 125 µM, Neu5Ac at 25 µM, Fuc, GalN and Glc at 1 mM (Table 4).
Results shows lectin activity with recognition in O group with high specificity to GlcNAc, GalNAc in both Eichornia crassipens and Lemna minor p-ext and also highly specificity to Neu5Ac recognition in Lemna minor p-ext. The results indicate that both p-ext displayed a common GlcNAc/GalNAc and also a Neu5Ac/GalNAC lectin activity similar as other type lectins reported [19].
Effect of the p-exts on the hemolytic activity of Vc
Lemna minor and Eichornia crassipens p-ext protect the group O of the hemolytic activity of Vibrio cholerae at titer of 1:4 and 1 : 64, respectively, using the hemolysin of Vibrio cholerae at titer of 1 : 128 as the materials and methods (Table 5).
Both p-exts contain lectins capable to recognize Fuc. Due to Fuc is involved in the hemolytic activity Vct, we consider that the p-exts may have lectins which recognized either Fuc, Glc or GlcNAc in glycosidic chains present in the hemolysin or its receptor in group O, and they are different of the domain of the antigenic O group since the HA activity in that group was 1 : 128 for Lemna minor and 1 : 4 for Eichornia crassipens. Whereas the protective effect against hemolytic activity was 1 : 4 and 1 : 64, respectively. This means that Eichornia crassipens p-ext has a protein with more specific anti-hemolytic activity and Lemna minor p-ext has more recognition to group O.
Effects of root p-ext on Vc proliferation y conservation
Lemna minor and Eichornia crassipens p-ext produced an effect on Vct density at 1 : 4 and 1 : 8 titer around the disk (see Table 5), both has the same effect on increase proliferation. The other tested dilutions seem to have a bacteriostatic effect. These results are different on the environmental habitats of Vc because the reservoir capacity for Vc and parahaemolyticus is higher in Lemna minor than in Eichornia crassipens root [21], however the results in Table 2 shows that Vct has similar growth in both roots but has a longer periods of survival in Eichornia crassipens than in Lemna minor, where the roots disappear.
Effect of root p-ext on agglutination of Vct
Graph 1 shows that when was using 214 μgr/ml to Eichornia crassipens and 175 μgr/ml to Lemna minor in double dilutions serials added to 2% of Vc suspension, in both p-ext was a significant reduction of the OD at 214 µgr/mL p ≤ 0.01 and 10 µgr/mL p ≤ 0.05 with the Eichornia crassipens and Lemna minor p-exts respectivaly. The Graph only shows Eichornia crassipens results, Lemna minor has similar results. OD reduction is related to agglutination, likewise to polivalent serum compared to saline alone. However, we discovered that phosphate also reduced OD likewise to polyvalent serum, the OD significant reduction, probably belongs from phosphate agglutination or salt precipitation because at that concentration neither of both p-exts caused Vc agglutination (Figure 1).
DISCUSSION
The world has gone through several cholera pandemics. The cholera disease depends of behaves of the toxigenic vibrions, which can abruptly appear and disappear and letting the question behind: what happens to the Vc during those intervals? Most researches believed that Vc remains in interepidemics reservoirs.
The interepidemic reservoirs should be sites of survival and multiplication of pathogenic vibrions between epidemics that are not well known. During epidemics, toxigenic Vc can be isolated from local fresh water [25], but it disappears after the epidemic diminishes. Since vibrions normally inhabit oceans and estuaries during most of the year, there are no explanations of how the seasonality and endemicity of cholera are maintained, and how it can live in freshwater. Researchers have shown the association between vibrions and marine plants and plankton, and also are associated with freshwater plants cohabiting in the epidemic season [2, 5, 26, 27], In-vitro conditions Vc survive longer in association with Lemna minor that in freshwater.
Our results have demonstrated and corroborated the stability and parmanency of Vct on roots of Lemna minor and Eichornia crassipens after almost ten years following the last outbreaks an from first isolation [23]. In those isolation was showed the presence of gram negative bacteria attached to a thin mucin layer on Lemna minor roots [21, 22]; this layer may contain lectins, where the lectins probably serve as an adhesive factor as well as nutriment, and may be Chi or Rip type; by its high recognition to GlcNAc, Neu5Ac and GalNAc, probably the lectins from plants recognized those suggars on the Vc membrane composition or in the EPS. Furthermore, Vc also may recognize a glycoprotein or GlcNAc derivates on the roots plants which contained hemicelluloses and pectin polysaccharides [24, 25]. The specificity of Vc to GlcNAc derivates used as source of energy is well known [6, 7], also the adhesion, survival, and proliferation of vibrions on the surface of small crustaceans and on aquatic plants. The vibrions species produce chitinase or mucinase responsible for the degradation of chitin or mucin into soluble oligosaccharides or monosaccharides, which become sources of carbon and nitrogen in this way the vibrions return to the ecosystem biologically useful forms from insoluble polysaccharides to soluble sugars this could be the reason to keep in commensal or symbiotic association with those plants.
Therefore, the study of interactions between vibrions and surfaces containing GlcNAc or mucin is important for their impact on human health and their ecological significance. The way how the same bacterial surface binding to compound (GlcNAc) may have a dual function in the Vibrio cholerae life cycle, mediating the attachment to inanimate or animate substrates in the environment and to cell membranes in the intestinal tract and after discovery the biofilm formation this association is more clear. During these interactions, changes from hydrophilic to hydrophobic conditions may occur, which it could be associated with their lectin activity also with an induction of some catalytic function involved in the association, invasion and virulence. It is reported that in a biofilm the development of gradients can create anoxic and acidic zones in the interior of biofilm clusters.
At these proteins concentration, the lectins from plants did not agglutinate Vc despite that this bacterium may have GlcNAc in its membrane as part of the antigen O1 in the LPS, similar to the O1 antigen from Salmonella thyphimurium (galactose-glucose – Rhamnose-Mannose – abequose-OAc-GlcNAc), which shares with antigenic groups of Vc because cross immunity with the typhoid has been reported [28]. It is possible that those lectins may recognizing GlcNAc in EPS composition in order to attachment in them and help to the development the biofilm, or also probably, those lectins recognize GlcNAc in membrane Vibrio cholerae and allowed the adhesion. It is probably that after the association through either mechanism, 2 events may occur: one stimulates the mucinase, chitinase or perhaps pectinase Vibrio cholerae activity in order to obtain nutritional factors; and the second, the promotion of biofilm development to survival in extreme conditions, such as protection from oxidative stress and an acquired resistance to osmosis, including changes in pH, salinity, and oxidative environments and provides adaptive advantages for aquatic organisms.
The results of this work suggest that, perhaps the lectins present in roots of plants, could play an important role in the balance of Vc in them by controlling the proliferation or death in order to obtain the extra-DNA (eDNA) required to maintain the biofilm matrix [11, 12] and thus keep the Vc viable and cultivable form such as in Eichornia crassipens. On the contrarily with the roots of Lemna minor, these results may be due to the loss of nutrient, protease activity or a toxic lectin inhibited its growth and in the case of Eichornia crassipens roots may favored the biofilm formation and keep in a viable and cultivable form the Vc. But it is also possible that Vc form part of the intracellular biota of the macrophytes to maintain the sources of carbon and nitrogen.
These events may be involved in the ability to act as permanent reservoirs and may also have a role in transforming toxigenic Vct, mainly because the filamentous phage that carrying genes toxigenic, is coexisting in those environments, and finally climatological factors such as high temperatures followed by rains trigger the destruction of the biofilm and the dissemination of Vc, the biofilms are involved in nutrition acquisition, detachment and desemination of Vc, important factors in epidemiology of the cholera.
The plant-vibrion association may be involved in the Vc survivival, pathogenesis, life cycle and the epidemiological periodicity in places where this is endemic mainly in about 50 development countries, because those plants are fecal contamination indicator into water. However the commensal association between those plants with Vc should be considered and studied extensively in ecological terms and not only from the perspective of health.
Conflict of interest
The authors declare no conflict of interest.
The editorial office was on 19th October 2017.
Corresponding author:
Coutiño-Rodríguez R., MD
Instituto de Salud Pública, Universidad Veracruzana
Av. Luis Castelazo Ayala s/n. Col. Industrial Ánimas
91 190, Xalapa, Veracruz, México
E-Mail: ecoutino@uv.mx
Zdroje
1. Islam MS, Drasar BS, Sack RB. The aquatic flora and fauna as reservoirs of Vibrio cholerae: a review. J Diarrhoeal Dis Res 1994;12(2):87–96.
2. Islam MS, Drasar BS, Bradley DJ. Attachment of toxigenic Vibrio cholerae 01 to various freshwater plants and survival with a filamentous green alga, Rhizoclonium fontanum. J Trop Med Hyg 1989;92(6):396–401.
3. Vezzulli L, Guzman CA, Colwell RR, et al. Dual role colonization factors connecting Vibrio cholerae’s lifestyles in human and aquatic environments open new perspectives for combating infectious diseases. Curr Opin Biotechnol 2008;19(3):254–259.
4. Rosas EC, Solano I, Delgado M, et al. Vibrio cholerae: Una bacteria ambiental con diferentres tipos de vida. In: Microbiologia Ambiental, Rosas I, Cravioto, A., Escurra (Eds). Secretaria del Médio Ambiente y recursoso Naturales, Instituto de Ecología y Programa Universitario Medio Ambiente-UNAM, México D.F 2004:47–65.
5. Islam MS, Drasar BS, Sack RB. The aquatic environment as a reservoir of Vibrio cholerae: a review. J Diarrhoeal Dis Res 1993;11(4):197–206.
6. Pruzzo C, Vezzulli L, Colwell RR. Global impact of Vibrio cholerae interactions with chitin. Environ Microbiol 2008;10(6):1400–1410.
7. Tarsi R, Pruzzo C. Role of surface proteins in Vibrio cholerae attach-ment to chitin. Appl Environ Microbiol 1999;65(3):1348–1351.
8. Harris JB, Larocque RC, Qadri F, et al. Cholera. Lancet 2012;379(9835):2466–2476.
9. Borroto J. Ecology of Vibrio cholerae serogroup O1 in aquatic environments. Rev. Panamericana de salud Pública/ Pan Am J. Public Health 1997;2(5):328–333.
10. López D, Vlamakis H, Kolter R. Biofilms. Cold Spring Harb Perspect Biol 2012;2(7): a000398.
11. Seper A, Fengler VH, Roier S, et al. Extracellular nucleases and extracellular DNA play important roles in Vibrio cholerae biofilm formation. Mol Microbiol 2011;82(4):1015–1037.
12. Karatan E, Watnick P. Signals, regulatory networks, and materials that build and break bacterial biofilms. Microbiol Mol Biol Rev 2009;73(2):310–347.
13. Kierek K, Watnick PI. Environmental determinants of Vibrio cholerae biofilm development. Appl Environ Microbiol 2003;69(9):5079–5088.
14. Watnick PI, Kolter R. Steps in the development of a Vibrio cholerae El Tor biofilm. Mol Microbiol 1999;34(3):586–595.
15. Wai SN, Mizunoe Y, Takade A, et al. Vibrio cholerae O1 strain TSI-4 produces the exopolysaccharide materials that determine colony morphology, stress resistance, and biofilm formation. Appl Environ Microbiol 1998;64(10):3648–3655.
16. Yildiz FH, Schoolnik GK. Vibrio cholerae O1 El Tor: identification of a gene cluster required for the rugose colony type, exopolysaccharide production, chlorine resistance, and biofilm formation. Proc Natl Acad Sci U S A 1999;96(7):4028–4033.
17. Wadstrom T, Trust DE. Bacterial superface lectins. Walter de Gruyter & Co., Berli, New York, 1983:342.
18. Coutino-Rodriguez R, Hernandez-Cruz P, Giles-Rios H. Lectins in fruits having gastrointestinal activity: their participation in the hemagglutinating property of Escherichia coli O157:H7. Arch Med Res 2001;32(4):251–257.
19. Van Damme JM, Pneumans WJ, Pusztai A, et al. In Handbook of Plants Lectins: Properties and biomedical aplications. New York Wiley and Sons, 1998.
20. Fisher W. Lectin content and specific in serum and mucus of oyster. J Shellfish Res 1991;10(1): 274.
21. Atzin J. Reservorios naturales de Vibrio cholerae y Vibrio parahaemolyticus. Congreso Nacional de Microbiología, 1998:57.
22. Coutiño-Rodríguez R. Lemna minor y Eichornia crassipens reservorios of Vibrio cholerae El Tor Inaba: Papel de las Lectinas en dicha asociación. Simposio Nacional del Cólera 2005:1.
23. Cordoba-Aguilar E, Herrera Rivero M, Rubi A, et al. Isolation of Vibrio cholerae El Tor Inaba From Lemna minor and Eichhornia crassipens Roots in Veracruz, Mexico. Jundishapur J Microbiol 2014;7(3):e6855.
24. Jesudason MV, Balaji V, Mukundan U, et al. Ecological study of Vibrio cholerae in Vellore. Epidemiol Infect 2000;124(2):201–206.
25. Khan MU, Shahidullah MD, Haque MS, et al. Presence of vibrios in surface water and their relation with cholera in a community. Trop Geogr Med 1984;36(4):335–340.
26. Islam MS. Seasonal rhythm of airway resistance and intrathoracic gas volume in healthy females and males. Respiration 1981;42(3):193–199.
27. Islam MS, Drasar BS, Bradley DJ. Survival of toxigenic Vibrio cholerae O1 with a common duckweed, Lemna minor, in artificial aquatic ecosystems. Trans R Soc Trop Med Hyg 1990;84(3):422–424.
28. Nikaido H, Vaara M. Outer membrane in Escherichia coli and Salmonella thyphimurium. Washington D.C. Neidhardd C Frederick et al., 1987.
Štítky
Hygiene and epidemiology Medical virology Clinical microbiologyČlánok vyšiel v časopise
Epidemiology, Microbiology, Immunology
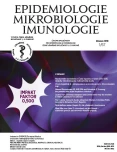
2018 Číslo 1
Najčítanejšie v tomto čísle
- A new definition of sepsis (Sepsis-3) – aims, advantages, and controversies
- Nosocomial candidemia in the Czech Republic in 2012–2015: results of a microbiological multicentre study
- West Nile virus (lineage 2) in mosquitoes in southern Moravia – awaiting the first autochthonous human cases
- Viral hepatitis A – seroprevalence and vaccine coverage rate in the South Moravian Region