Gut microbiota and autism spectrum disorders
Střevní mikrobiota a poruchy autistického spektra
Poruchy autistického spektra (PAS) se řadí mezi neurovývojové a neuropsychiatrické poruchy s klinickou manifestací v dětském věku charakterizované obtížemi v sociální interakci a komunikaci, limitovanými zájmy a repetitivními prvky v chování. V posledních letech došlo k významnému nárůstu prevalence PAS, aktuálně postihuje 1–2 % dětí. V etiopatogenezi onemocnění se uplatňují genetické faktory, určitou roli hrají i faktory prostředí. U celé řady pacientů s PAS jsou přítomny rozmanité gastrointestinální obtíže, především zácpa nebo růjem, plynatost nebo nespecifické bolesti břicha. Spojitost mezi těmito obtížemi a symptomy PAS se dostává v posledních letech do popředí vědeckého zájmu, a to především z hlediska vývoje nových molekulárně-biologických metod, které zkoumají složení střevní mikrobioty. Komunikace mezi střevem a CNS (tzv. osa střevo-mozek) je umožněna vysoce komplexním obousměrným neurohumorálním komunikačním systémem. Tento systém umožňuje působení střevní mikrobioty na mozkové funkce a umožňuje, aby mozkové signály ovlivňovaly aktivitu střevní mikrobioty a funkce gastrointestinálního traktu. Autoři shrnují různé patofyziologické mechanizmy zapojené do těchto procesů a jsou detailně diskutovány i rozličné terapeutické modality, které zasahují do složení a funkce střevní mikrobioty, jako je užívání vankomycinu, oxytocinu, prebiotik a probiotik. Jsou shrnuty i dosavadní poznatky z oblasti indikace a účinnosti fekální transplantace u dětí s PAS.
Klíčová slova:
autismus – poruchy autistického spectra – střevní mikrobiota – probiotika – fekální transplantace
Authors:
P. Danhofer 1; O. Horák 1; L. Knedlíková 1; S. Kolář 1; A. M. Bittnerová 1; P. Jabandžiev 2; H. Ošlejšková 1
Authors place of work:
Department of Pediatric Neurology, Masaryk University and University, Hospital, Brno, Czech Republic
1; Department of Pediatrics, Masaryk, University and University Hospital, Brno, Czech Republic
2
Published in the journal:
Cesk Slov Neurol N 2021; 84/117(2): 127-134
Category:
Přehledný referát
doi:
https://doi.org/10.48095/cccsnn2021127
Summary
Autism spectrum disorders (ASD) are neurodevelopmental and neuropsychiatric disorders with clinical manifestation in childhood, characterized by difficulties in social interaction and communication, limited interests, and repetitive behavior. In recent years, there has been a significant increase in the prevalence of this disorder, currently affecting 1–2% of children. In pathophysiology, genetic factors are mainly used, which are also modified in their phenotypic expression by environmental factors. A large percentage of patients with ASD also have a variety of gastrointestinal problems; children experience constipation or diarrhea, flatulence, or nonspecific abdominal pain. In last years, the connection of intestinal symptomatology with the manifestations of ASD has come to the forefront of scientific interest with the development of new molecular-biological methods of studying the community of intestinal microorganisms, the gut microbiota. Communication between the gut and the CNS (the gut-brain axis) is made possible by a very complex two-way neurohumoral communication system. This system ensures the effect of the intestinal microbiota on brain functions and allows the brain signals to affect the intestinal microbiota activity and the gastrointestinal tract functions. This article focuses on various pathophysiological mechanisms involved in these processes and discusses in detail the possible therapeutic modalities that affect the gut microbiota composition and function, such as the use of vancomycin, oxytocin, prebiotics and probiotics, as well as fecal transplantation, which has very promising results in relation to both gastrointestinal and ASD symptoms.
Keywords:
Autism – autism spectrum disorders – gut microbiota – probiotics – fecal transplantation
Introduction
Autism spectrum disorders (ASD) are neurodevelopmental and neuropsychiatric disorders with clinical manifestation in childhood, characterized by difficulties in social interaction and communication, limited interests, and repetitive behavior. Symptoms persist throughout the life; children with ASD switch to adult care after the age of 18. ASD are more common in men at a 4 : 1 ratio. The estimated prevalence of ASD in the population increased from 1 out of 476 to 1 out of 323 in the 1990s [1,2] to 1 or 2 out of 100 in 2014 [3,4]. In about half of the patients, the higher incidence can be explained by better diagnosis and awareness of ASD, older age of parents, and geographical groupings of families; in the remaining patients, the cause of the increased prevalence of ASD is unclear [5].
The pathophysiological mechanisms involved in the development of ASD remain a subject of intensive research. Genetics play an important role; a number of candidate genes involved in the pathophysiology of ASD have been identified [6]. At the current level of knowledge, the most widespread theory is the genetic predisposition of an individual, where various environmental factors can influence or trigger psychiatric symptoms. Such a “non-genetic but hereditary” factor may be the composition and diversity of the gut microbiota, a highly structured community of all the microorganisms that inhabit the intestine of each individual.
The link between the composition of the gut microbiota and the development of ASD symptoms seems very unlikely at first glance. However, it is clear from clinical practice that children with ASD have very often digestive problems and various gastrointestinal tract symptoms, such as abdominal pain, persistent constipation or, conversely, diarrhea, flatulence, etc. [7].
Recent studies show that the gut microbiota is involved, through a number of mechanisms, in a very important communication channel, the gut-brain axis, which will be described below. Normal colonization patterns of the gut microbiota are very important for the proper development and function of the CNS; they provide key homeostatic mechanisms in the development of inflammatory processes. Intestinal microbial dysbiosis may be associated with the development or worsening of ASD symptoms.
Normal gut microbiota
The human gut contains up to 80–100×1012 microorganisms [8], which in total have about 100times more genes than the human genome [9]. The gut microbiota is not only made up of bacteria (bacterioma), but also of a whole range of viruses, fungi, and other microorganisms. The gut is thought to colonize 40,000 different species of bacteria, the analysis of which is currently based on the detection of their specific 16S (18S) ribosomal ribonucleic acid sequences [10]. It is possible to cultivate less than 3,000 bacterial species from the human body by culture [11]. It is therefore indisputable that molecular-biological methods leading to the discovery of a considerable diversity of gut microbiota have provided new knowledge and opened a number of questions about the composition, function, and significance of this community.
The microbial population pattern is unique for each individual and is based on genetic predisposition [10] and influenced by a number of environmental factors. The management of childbirth can have a significant effect on the composition of the microbiota. Cesarean section delivery does not allow the baby to come into contact with the mother’s vaginal microbiota and to acquire non-physiological microbial stimuli, especially from the skin. Nutrition and a type of diet also have an undeniable influence on microbiota composition [10]. Breastfeeding significantly positively affects its composition, as breast milk is a source of probiotic oligosaccharides; plants are a source of soluble prebiotic fiber; and important microorganisms such as “probiotics” are found in fermented dairy products [10]. Animal products do not have such benefits for the microbiota. Industrially processed foods treated by methods leading to the removal of pathogenic microorganisms and using a wide range of chemicals have an undeniably negative impact.
Human physiological microbiota undergoes relatively dynamic advancement during ontogenetic development. The dominant gut microbial phyla are Firmicutes, Bacteroidetes, Actinobacteria, Proteobacteria, Fusobacteria, and Verrucomicrobia, with the two phyla Firmicutes and Bacteroidetes representing 90% of the gut microbiota. The Firmicutes phylum is composed of more than 200 different genera, such as Lactobacillus, Bacillus, Clostridium, Enterococcus, and Ruminicoccus. Bacteroidetes consists of predominant genera, such as Bacteroides and Prevotella. The Actinobacteria phylum is proportionally less abundant and mainly represented by the Bifidobacterium genus [12]. Significant differences in the composition of the gut microbiota can be observed between premature and full-term neonates. In premature infants, two essential bacterial genera are missing: Bifidobacterium and Lactobacillus with a compensatory predominance of Proteobacteria phylum [13,14]. The infant digestive tract is populated by microorganisms from the Actinobacteria and Proteobacteria phyla [10]. In adults, the highest proportion of bacteria in the gut microbiota is formed by the Bacteroidetes, which includes the genera Bacteroides and Prevotella. Adult intestinal bacteria include the phylum Firmicutes, which includes the genera Clostridium and Lactobacillus. Bacteria of the Proteobacteria and Actinobacteria phyla are also found in adult intestinal bacteria [10,15].
The microbial population pattern is partly genetic but is influenced by a number of external factors and is thus highly individual. For example, geographical differences in microbiotic composition are so significant that small samples of subpopulations from a particular geographical area are highly heterogeneous and therefore healthy controls cannot be easily defined, even in a relatively homogeneous population in terms of genetics, nutritional habits, and ethnicity. In their research, He et al studied gut microbiota in China’s Guangdong Province with a population of 108 million. They randomly selected 14 districts in the province and analyzed samples from 7,009 individuals over the age of 18. They found that the geographical differences in the composition of the intestinal microbiota exceeded those differences that were evident when comparing samples from “healthy” individuals with individuals experiencing various metabolic diseases (type 2 diabetes mellitus, metabolic syndrome, and obesity). The authors believe that this explains the origin of inconsistent dysbiotic patterns observed in studies on small population samples [16].
In terms of “healthy” microbiota, not only its specificity but also the diversity and number of individual bacterial species are certainly important. Studies comparing the microbiota populations of Western civilizations with those of indigenous tribes show a significant reduction in bacterial diversity in modern conditions, and this trend is supported by globalization [17–19].
Mechanisms of communication on the gut-brain axis
Communication between the gut and the CNS (the so-called gut-brain axis) is made possible by a very complex two-way neurohumoral communication system (Fig. 1). This system ensures the action of the gut microbiota on brain functions and allows the brain signals to affect the microbiota activity and gastrointestinal tract functions. The autonomic nervous system plays an important role here. During stress reactions, the sympathetic system activity is increased and intestinal functions, specifically intestinal motility and secretion, are inhibited. With long-term stress, sympathetic hyperactivity occurs to such an extent that intestinal integrity is damaged, intestinal epithelium is destroyed, and intestinal motility and secretion are impaired. These changes lead to a change in the gut microbiota composition and to the development of pathological inflammatory processes [20,21]. Stressed mice have been shown to have a hyperactive hypothalamic-pituitary-adrenal axis, leading to increased serum cortisol and proinflammatory cytokines and to the development of intestinal inflammation [22]. The effects of stress leading to dysbiosis of the gut microbiota are well documented, for example, in irritable bowel syndrome [23].
ANS – autonomic nervous system; ASD – autism spectrum disorders; BBB – blood-brain barrier; CHD8 – chromodomain helicase DNA-binding protein
8; GABA – gamma aminobutyric acid; GIT – gastrointestinal tract; HDAC – histone deacetylase; IL – interleukin; SCFA – short-chain fatty acids
Obr. 1. Osa střevo-mozek a poruchy autistického spektra. Obrázek ilustruje možné cesty, kterými jsou střevo a mozek spojeny u zdravých jedinců,
jedinců s PAS a u myší s transplantovanou střevní mikrofl órou od jedinců s PAS. Chronický stres zvyšuje aktivitu sympatického systému,
což poškozuje intestinální integritu, to vede ke snížení střevní motility a sekrece. Střevní mikrobiota produkuje SCFA, které snižují produkci prozánětlivých
cytokinů (IL-1β, IL-6 a TNF-a) a zvyšují produkci protizánětlivých cytokinů. SCFA mohou také pronikají HEB a inhibují HDAC. Některé
druhy bakterií produkují neurotransmitery (serotonin, GABA, norepinefrin atd.), které mohou pronikat přes HEB. Nadměrný růst druhů r. Candida
ve střevní mikrofl óře u pacientů s PAS může nepříznivě ovlivnit funkci GIT v důsledku dysregulace cytokinů. Mutace v genu CHD8 identifi
kovaném jako kandidátní gen v patogenezi PAS poškozuje střevní motilitu. Bakteriálně naivní myši s transplantovanou střevní mikrofl órou od
lidských dárců s PAS vykazovaly znaky autistického chování, pravděpodobně v důsledku snížené produkce střevních agonistů GABAA-receptoru,
kyseliny 5-aminovalerové a taurinu. Obrázek byl vytvořen pomocí BioRender (San Francisco, CA, USA).
ANS – autonomní nervová soustava; GABA – kyselina γ-aminomáselná; GIT – gastrointestinální trakt; HDAC – histonová deacetyláza; HEB – hematoencefalická
bariéra; CHD8 – chromodoménový helikázový DNA-vazebný protein 8; IL – interleukin; PAS – poruchy autistického spektra;
SCFA – mastné kyseliny s krátkým řetězcem
The results of recent research show that the effect of gut microbiota far exceeds the intestinal boundary. The bacterial gut microbiota is able to produce various metabolites that are applied at several levels. They are able to maintain the integrity of intestinal epithelia, affect nutrient resorption and fat metabolism, and affect intestinal motility. For example, short-chain fatty acids (SCFAs) play a crucial role. Bacteria obtain energy and produce metabolites, particularly from SCFAs (especially acetic, propionic, and butyric acid), by fermenting indigestible carbohydrates (e. g., cellulose, xylan, inulin) [24]. These metabolites are able to reduce in vitro production of proinflammatory cytokines interleukins (IL-1â, IL-6), and tumor necrosis factor-alpha (TNF-á). In contrast, they increase the production of anti-inflammatory cytokines, such as IL-10 [25]. SCFAs are also able to penetrate the blood-brain barrier (BBB) and exert their functions in the brain, either directly or indirectly through the G-protein coupled receptors. Butyric acid, for example, exerts its function in the brain as an epigenetic modulator through histone deacetylases [25–27].
The gut microbiota is able to regulate key central neurotransmitters at the level of their precursors. SCFAs are able to increase the production of enteral serotonin and ensure its homeostasis. Monoamines such as norepinephrine, dopamine, and serotonin can also be produced by the gut microbiota. Certain types of bacteria are able to modulate serotonin secretion from colonic enterochromaffin cells and modulate its concentration in both the intestine and the blood [25]. Bifidobacterium infantis increases plasma tryptophan levels and thus affects central serotonergic transmission [28]. Bacteria can produce neurotransmitters themselves, as has been shown in Lactobacillus and Bifidobacterium genera, which produce gamma aminobutyric acid (GABA). Animal studies evidenced that Lactobacillus can modulate the levels of serotonin and dopamine in the brain, influencing early brain development [29]. Escherichia, Bacillus, and Saccharomyces in turn produce norepinephrine. Escherichia can also produce serotonin and Bacillus dopamine [28]. These bacterially synthesized neurotransmitters are able to pass through the intestinal mucosa and exert their physiological functions in the brain.
Pathogenic gut microbiota leads to the stimulation of the secretion of proinflammatory cytokines (IL-1â, IL-6, IL-18) through intestinal epithelial and dendritic cells. These lead to the development of gut inflammation and increased intestinal permeability and are associated with various neuropsychiatric diseases, such as depression, anxiety, and schizophrenia [25].
Gut microbiota and gut-brain communication in ASD
There are certainly several reasons that the connection between the intestine and the symptoms of autism has been studied. The symptoms of gastrointestinal disease have long been observed in children with ASD, as mentioned in the introduction. Gastrointestinal problems are observed 6–8× more often in children with autism than in the general population [7]; interestingly, the severity of symptoms correlates with the severity of autism [7]. In rare cases, this connection can also be explained genetically. For example, chromodomain helicase DNA-binding protein 8 (CHD8) is identified as a candidate gene in the pathogenesis of ASD. Children with this mutation had a much higher rate of constipation than other patients with ASD (up to 60% of cases). Studies have shown that mutations in CHD8 gene damage the intestinal motility by interfering with neuronal colonization of the gastrointestinal tract [30].
The presence of gastrointestinal tract symptoms in children with ASD in itself encourages the study of pathological processes in the gut in these patients in relation to the severity of the clinical expression of the disorder, also because it opens various therapeutic options; due to the absence of any causal treatment, such options are very tempting in children with ASD. However, a deeper study of this methodology presents numerous obstacles. One very fundamental problem is the definition of “healthy control” in relation to the composition of the intestinal microbiota. The gut microbiota has an individual genetic imprint for each individual and is influenced by a number of factors, from the type of birth control, through breastfeeding to pharmacological influences, nutritional factors, stress, and other environmental factors, including geographical variability. Creating a “normal gut microbiota map” is a difficult task. Although it is possible to create a relatively sophisticated map of its composition with the help of molecular genetic methods, its interpretation is not easy [16].
Children with ASD have a lower level of Akkermansia, Bacteroides, Bifidobacterium, Escherichia, and Enterococcus, a higher level of Faecalibacterium, and slightly increased level of Ruminococcus and Clostridium. Reduced levels of beneficial bacteria combined with the increased levels of harmful bacteria contribute together to ASD symptoms [31]. Several studies showed that there was a higher level of Clostridium in the individuals with ASD compared to controls and hypothesized that Clostridium can produce neurotoxins and contributed to ASD [31]. A higher level of Bifidobacterium negatively correlates with ASD symptoms. Bifidobacterium is among first colonizers of the human gut and one of the dominant groups in the gut microbiota of breast-fed infants. It can ferment complex polysaccharides to regulate host functions and promote health, encouraging the interest in its use as a probiotic [32].
Strati et al observed a significantly higher Firmicutes/ Bacteroidetes ratio in children with ASD, due to a significant reduction in Bacteroidetes phylum in these children [33]. Bacteriodetes and its genus Bacteroides are the main producers of propionate in the gut, and the abundance of propionate in feces correlates strongly with the abundance of Bacteroides. Propionate produced by the microbiota is used for gluconeogenesis in the liver and represents a source of glucose in the host. The study indicated that neurodevelopmental abnormality in ASD patients is accompanied with impaired propionic acid metabolism, which may relate to the change of propionate-producing bacteria [34]. The results in mouse models show that the enhancement of Bacteroides fragilis sp. can lead to altered gut microbiota and blood metabolite profile, modify intestinal permeability, and improve ASD behavior [35]. The elevated Proteobacteria phylum was found in ASD patients. It is noted that Proteobacteria is a major gram-negative bacteria and includes a variety of opportunistic pathogens. Meanwhile, as microbial signature of dysbiosis in the gut microbiota, Proteobacteria is associated with host inflammation. These gram-negative bacteria produce a potent toxic factor lipopolysaccharide (LPS). An animal study indicated that prenatal LPS exposure reduced the level of glutathione in the brain. Glutathione is a significant antioxidant, closely related to detoxification in the brain [32]. It was found that in patients with ASD, Candida yeast is twice as present in the gut microbiota as in the control group. The interpretation of these results is difficult, and the authors hypothesize that alterations in the intestinal fungal population due to Candida overgrowth in the gut microbiota in children with ASD may adversely affect the gastrointestinal function due to cytokine network dysregulation [33].
Thus, the representation of individual bacterial genera and their taxonomic description will play an important role, but so will the mechanisms by which individual bacteria are involved in various neurohumoral, neurometabolic, and neuroimmunological processes. Immune dysregulations in patients with ASD were observed, including alterations in circulating and brain cytokines, chemokines, and other inflammatory factors, as well as abnormal distributions and/ or reactivity of various leukocyte subtypes. Certain inflammatory cytokines (i.e., interferon- ã, IL-1ß, IL-6, IL-12, TNF-á) may be associated with ASD symptoms and their severity. Increased levels of some chemokines (i.e., CCL-2, CCL-5) are associated with higher abberant behavioral scores and more impairments in the development [36].
A number of studies have dealt with the gut microbiota in relation to many major diseases in any organ system; the list is beyond the scope of this text, which is focused only on patients with ASD. The crucial question is whether the differences in the gut microbiota are causal in relation to ASD, or whether they have a mere association between the two diseases [10]. It has been suggested that the changes in the gut microbiota composition may be caused by limited nutritional patterns in these patients, leading to the reduction in bacterial diversity and overgrowth of other strains. The gut microbiota of patients with ASD has an as yet unspecified but characteristic pattern, as shown by the results of a recently published study [37] in which the authors transplanted the gut microbiota from human donors with ASD to germ-free mice and found that these mice expressed autistic behavior traits. The authors performed a metabolomic analysis of the intestinal contents of mice with ASD and a control group. They found that the differences in GABA and glycine receptor inhibitory agonists and antagonists could be detected in autistic mice. In particular, they found 5-aminovaleric acid (5-AV), a fermentation product of proline, which is considered a weak agonist of GABAA receptors with anticonvulsant effects in mice and is significantly lower in patients with ASD [37]. They also showed lower levels of taurine, a metabolic product of taurocholic acid that is essential for brain development [38], is produced by neurons and astrocytes, and is transported across the BBB by the taurine transporter TauT. It is a weak GABAA agonist and also a possible glycine receptor agonist that is reduced in patients with ASD [7].
The results support the theory that gut bacteria may affect inhibitory GABA signaling. Some forms of ASD are thought to be caused by excitatory-inhibitory imbalances in synaptic transmission and involvement in corticosubcortical circuits [6]. The possibilities of therapeutic intervention in this imbalance at the level of influencing synaptic transmission are the subject of studies and have been shown to be effective in mouse models [39–41]. The authors [37] tested whether 5-AV and taurine affect the cortical circuits and interfere with the excitatory-inhibitory balance by registering the activity from fifth-layer pyramidal neurons in the medial prefrontal cortex. Specifically, they examined whether the treatment with 5-AV and taurine from conception to adulthood affected the membrane and synaptic properties of pyramidal neurons (miniature inhibitory or excitatory postsynaptic potentials). Pyramidal neurons from the fifth cortical layer were shown to have significantly lower excitability in 5-AV-treated mice. GABA excitation is thought to shift physiologically to GABA inhibition during the development, and this signaling change does not occur in children with ASD, which is thought to be one of the possible mechanisms in the pathophysiology of autism [6]. Taurine is an inhibitor of the potassium chloride cotransporter KCC2 [42] and can thus interfere with the excitatory-inhibitory switching in GABAergic transmission, thereby modulating neuronal development and the response of neurons to inhibitory neurotransmitters. In summary, the gut microbiota can influence complex behavior in mice through the formation of neuroactive microbial metabolites using the mechanisms that interfere with the regulation of excitatory-inhibitory balance in the brain [37].
Therapeutic options
Influencing the symptoms of autism by interfering with the gut microbiota of patients has been a subject of research for 20 years (Tab. 1). The first studies were performed using the non-absorbable antibiotic vancomycin, which may have a short-term effect on the symptoms of ASD. This has been demonstrated in small groups of autism patients treated with vancomycin for 8 weeks [43,44]. Increased attention is currently being paid to oxytocin, a hypothalamic peptide that increases sociability. Mice with blocked oxytocin receptors show a significant deficit in social behavior [45]. Interestingly, the probiotic bacterium Lactobacillus reutri is able to influence the activity of the posterior hypothalamus and increase the level of oxytocin, which makes it possible to intervene in social behavior through the influence of gut microbiota [46]. Preclinical studies show that intranasally administered oxytocin can positively influence social behavior patterns [46].
A number of studies have addressed the use of prebiotics and probiotics in the treatment of ASD symptoms. These are mostly prospective studies, open-label or double-blind, with 8–30 participants in the ASD and control groups. Prebiotics (colostrum, carrot powder, guar gum, vitamin A) combined with probiotics (various genera of Lactobacillus and Bifidobacterium) were given for 2–12 months. The results show that there may be a reduction in negative gastrointestinal symptoms [47–49], and in some there may be a statistically significant effect on behavioral disorders within ASD [47,48,50]. However, prebiotics and probiotics often have no effect on gastrointestinal tract symptoms or ASD symptoms [51,52]. In summary, the results of the studies are generally not very encouraging and the effects of prebiotics and probiotics on the reduction of ASD symptoms are small. Inoue et al demonstrated a statistically significant reduction in microbial diversity and levels of some cytokines (IL-1â, IL-6 and TNF-á) after prebiotic administration [50]. Another study showed a statistically significant increase in the Bacteroidetes/ Firmicutes ratio after vitamin A supplementation, but without the evidence of the improvement in ASD symptoms [51].
Compared to probiotics, which contain only a few bacterial strains from dairy cultures, the method of fecal transplantation (microbiota transfer therapy; MTT) is very interesting from a therapeutic point of view, as thousands of natural gut bacterial species can be administered at once. This therapy is effective in the treatment of recurrent Clostridium difficile infections and also yields promising results in the treatment of inflammatory bowel disease [52–54]. Kang et al performed MTT in 18 children with ASD aged 7–16 years in a prospective open-label study [55]. Children received oral vancomycin for 14 days before transplantation, followed by 12–24 h of fasting to clean the bowels. Thereafter, children received a high-dose initial dose of standardized human gut microbiota (SHGM) either rectally or orally followed by daily oral maintenance low-dose SHGM therapy in combination with proton pump inhibitors for 7–8 weeks. There was an improvement in the gastrointestinal symptoms scale of approximately 80% (P < 0.001) with a significant improvement in symptoms such as constipation, diarrhea, and abdominal pain. This improvement persisted for 8 weeks after stopping the treatment. Likewise, the manifestations of ASD improved in several psychological tests. Significant changes were observed in the results of the Childhood Autism Rating Scale (CARS), a diagnostic tool used in children with ASD. The CARS score decreased by 22% at the end of the treatment and by a further two percent in 8 weeks after the end of the treatment. There was an improvement in the Social Responsiveness Scale (SRS) score, which assesses deficits in the social sphere. The Vineland Adaptive Behavior Scale (VABS) score, which is used to assess adaptive behaviors such as communication, normal daily skills, and socialization, showed an increase of 1.4 years (P < 0.001) in all spheres. The results in these tests tended to further improve when evaluated in 8 weeks after the end of the treatment. The study of gut microbiota showed an overall improvement in microbial diversity with an increase in Bifidobacterium, Prevotella, and Desulfovibrium, again with an effect lasting even 8 weeks after the end of the treatment. Importantly, the authors did not show a significant difference in results when comparing the group where SHGM was administered rectally with the group with oral initial high-dose administration. A follow-up study by the same scientific team, which indicated the effect of MTT two years after its implementation, also showed very positive results [56]. Further favorable results were obtained in the study of 24 patients with ASD, where fecal transplantation led to an increase in the proportion of Bacteroides fragilis and Bacteroides vulgatus in feces and improved the composition of gut microbiota and ASD symptoms in relation to Coprococcus comes [57]. No adverse reactions or complications of fecal transplantation were reported in any of these studies and the transplantations were generally well tolerated. Although the results of the studies with MTT appear promising, the effect is only partial and proves that the pathophysiology of ASD is complex and further research concerning the involvement of the gut microbiota in the development of ASD is needed.
Conclusion
Given the growing prevalence of autism and the severity of this disorder, the question of therapeutic options is increasingly relevant. It is clear that the pathophysiology of ASD is complex, with both genetic and environmental influences. If the symptoms can be influenced by interfering with the composition of the gut microbiota, then this possibility is certainly a great challenge.
Acknowledgments
The publication was supported by funding through the Faculty of Medicine of Masaryk University for junior researcher Pavlína Danhofer, MD, PhD (ROZV / 28 / LF / 2020).
Conflict of interest
The authors declare they have no potential conflicts of interest concerning drugs, products, or services used in the study.
The Editorial Board declares that the manuscript met the ICMJE “uniform requirements” for biomedical papers.
Redakční rada potvrzuje, že rukopis práce splnil ICMJE kritéria pro publikace zasílané do biomedicínských časopisů.
Pavlína Danhofer, MD, PhD
Department of Pediatric Neurology
Masaryk University
University Hospital
Černopolní 9
613 00 Brno
Czech Republic
e-mail: pavlina.cahova@seznam.cz
Accepted for review: 4. 1. 2021
Accepted for print: 15. 4. 2021
Zdroje
1. Arvidsson T, Danielsson B, Forsberg P et al. Autism in 3-6-year-old children in a suburb of Goteborg, Sweden. Autism 1997; 1(2): 163–173. doi: 10.1177/ 1362361397012004.
2. Baird G, Charman T, Baron-Cohen S et al. A screening instrument for autism in 18 months of age: a 6-year-follow-up study. J Am Acad Child Adolesc Psychiatry 2000; 39(6): 694–702. doi: 10.1097/ 00004583-200006000-00007.
3. Yin J, Schaaf CP. Autism genetics – an overview. Prenat Diagn 2017; 37(1): 14–30. doi: 10.1002/ pd.4942.
4. Lai MC, Lombardo MV, Baron-Cohen S. Autism. Lancet 2014; 383(9920): 896–910. doi: 10.1016/ S0140-6736(13)61539-1.
5. Weintraub K. The prevalence puzzle: Autism counts. Nature 2011; 479(7371): 22–24. doi: 10.1038/ 479022a.
6. Danhofer P, Horák O, Aulická Š et al. Genetické a neurobiologické aspekty komorbidního výskytu poruch autistického spektra a epilepsie. Cesk Slov Neurol N 2019; 82/ 115(2): 148–154. doi: 10.14735/ amcsnn2019148.
7. Adams JB, Johansen LJ, Powell LD et al. Gastrointestinal flora and gastrointestinal status in children with autism – comparisons to typical children and correlation with autism severity. BMC Gastroenterol 2011; 11: 22. doi: 10.1186/ 1471-230X-11-22.
8. Bermon S, Petriz B, Kajeniene A et al. The microbiota: an exercise immunology perspective. Exerc Immunol Rev 2015; 21: 70–79.
9. Stilling RM, Dinan TG, Cryan JF. Microbial genes, brain and behavior – epigenetic regulation of the gut-brain axis. Genes Brain Behav 2014; 13(1): 69–86. doi: 10.1111/ gbb.12109.
10. Krejsek J. Roztroušená skleróza mozkomíšní, úloha střevní mikrobioty v poškozujícím zánětu. Cesk Slov Neurol N 2019; 82/ 115(2): 141–147. doi: 10.14735/ amcsnn2019141.
11. Bilen M, Dufour JC, Lagier JC et al. The contribution of culturomics to the repertoire of isolated human bacterial archaeal species. Microbiome 2018; 6(1): 94. doi: 10.1186/ s40168-018-0485-5.
12. Rinninella E, Raoul P, Cintoni M et al. What is the healthy gut microbiota composition? A changing ecosystem across age, environment, diet, and diseases. Microorganisms 2019; 7(1): 14. doi: 10.3390/ microorganisms7010014.
13. Barrett E, Deshpandey AK, Ryan CA et al. The neonatal gut harbours distinct bifidobacterial strains. Arch Dis Child Fetal Neonatal Ed 2015; 100(5): F405–F410. doi: 10.1136/ archdischild-2014-306110.
14. Barrett E, Guinane CM, Ryan CA et al. Microbiota diversity and stability of the preterm neonatal ileum and colon of two infants. Microbiologyopen 2013; 2(2): 215–225. doi: 10.1002/ mbo3.64.
15. Virtanen S, Kalliala I, Nieminen P et al. Comparative analysis of vaginal microbiota sampling using 16S rRNA gene analysis. PLoS One 2017; 12(7): e0181477. doi: 10.1371/ journal.pone0181477.
16. He Y, Wu W, Zheng HM et al. Regional variations limits applications of healthy gut microbiome reference ranges and disease models. Nature 2018; 24(10): 1532–1535. doi: 10.1038/ s41591-018-0164-x.
17. Leach J. Gut microbiota: please pass the microbes. Nature 2013; 504(7478): 33. doi: 10.1038/ 504033c.
18. Sonnenburg ED, Smits SA, Tikhonov M et al. Diet-induced extinctions in the gut microbiota compound over generations. Nature 2016; 529(7585): 212–215. doi: 10.1038/ nature16504.
19. Clemente JC, Pehrsson EC, Blaser MJ et al. The microbiome of uncontacted Amerindians. Sci Adv 2015; 1(3): e1500183. doi: 10.1126/ sciadv.1500183.
20. Al Omran Y, Aziz Q. The brain-gut axis in health and disease. Adv Exp Med Biol 2014; 817: 135–153. doi: 10.1007/ 978-1-4939-0897-4_6.
21. Collins SM, Surette M, Bercik P. The interplay between the intestinal microbiota and the brain. Nat Rev Microbiol 2012; 10(11): 735–742. doi: 10.1038/ nrmicro2876.
22. Sun Y, Zhang M, Chen CC et al. Stress-induced corticotropin releasing hormone-mediated NLRP6 inflammasome inhibition and transmissible enteritis in mice. Gastroenterology 2013; 144(7): 1478–1487. doi: 10.1053/ j.gastro.2013.02.038.
23. Dinan TG, Quigley EM, Ahmed SM et al. Hypothalamic-pituitary-gut axis dysregulation in irritable bowel syndrome: plasma cytokines as a potential biomarker? Gastroenterology 2006; 130(2): 304–311. doi: 10.1053/ j.gastro.2005.11.033.
24. Li Q, Zhou JM. The microbiota-gut-brain axis and its potential therapeutic role in autism spectrum disorder. Neuroscience 2016; 324: 131–139. doi: 10.1016/ j.neuroscience.2016.03.013.
25. Vinolo MA, Rodrigues HG, Nachbar RT et al. Regulation of inflammation by short chain fatty acids. Nutrients 2011; 3(10): 858–876. doi: 10.3390/ nu3100858.
26. Galland L. The gut microbiome and the brain. J Med Food 2014; 17(12): 1261–1272. doi: 10.1089/ jmf.2014.7000.
27. Paul B, Barnes S, Demark-Wahnefried W et al. Influences of the diet and gut microbiome on epigenetic modulation in cancer and other diseases. Clin Epigenetics 2015; 7: 112. doi: 10.1186/ s13148-015-0144-7.
28. Dinan TG, Cryan JF. Gut instincts: microbiota as a key regulator of brain development, ageing and neurodegeneration. J Physiol 2017; 595(2): 489–503. doi: 10.1113/ JP273106.
29. Yang J, Fu X, Liao X et al. Effects of gut microbial-based treatments on gut microbiota, behavioral symptoms, and gastrointestinal symptoms in children with autism spectrum disorder: a systematic review. Psychiatry Res 2020; 293: 113471. doi: 10.1016/ j.psychres.2020.113471.
30. Bernier R, Golzio C, Xiong B et al. Disruptive CHD8 mutations define a subtype of autism early in development. Cell 2014; 158(2): 263–276. doi: 10.1016/ j.cell.2014.06.017.
31. Finegold SM, Summanen PH, Downes J et al. Detection of Clostridium perfringens toxin genes in the gut microbiota of autistic children. Anaerobe 2017; 45: 133–137. doi: 10.1016/ j.anaerobe.2017.02.008.
32. Liu F, Li J, Wu F et al. Altered composition and function of intestinal microbiota in autism spectrum disorders: a systematic review. Transl Psychiatry 2019; 9(1): 43. doi: 10.1038/ s41398-019-0389-6.
33. Strati F, Cavalieri D, Albanese D et al. New evidence on the altered gut microbiota in autism spectrum disorders. Microbiome 2017; 5(1): 24. doi: 10.1186/ s40168-017-0242-1.
34. Wang L, Christophersen CT, Sorich MJ et al. Elevated fecal short chain fatty acid and ammonia concentrations in children with autism spectrum disorder. Dig Dis Sci 2012; 57(8): 2096–20102. doi: 10.1007/ s10620-012-2167-7.
35. Hsiao EY, McBride SW, Hsien S et al. Microbiota modulate behavioral and physiological abnormalities associated with neurodevelopmental disorders. Cell 2013; 155(7): 1451–1463. doi: 10.1016/ j.cell.2013.11.024.
36. Ashwood P, Krakowiak P, Hertz-Picciotto I et al. Associations of impaired behaviors with elevated plasma chemokines in autism spectrum disorders. J Neuroimmunol 2011; 232(1–2): 196–199. doi: 10.1016/ j.jneuroim.2010.10.025.
37. Sharon G, Cruz NJ, Kang DW et al. Human gut microbiota from autism spectrum disorder promote behavioral symptoms in mice. Cell 2019; 177(6): 1600–1618. doi: 10.1016/ j.cell.2019.05.004.
38. Tochitani S. Functions of maternally-derived taurine in fetal and neonatal brain development. Adv Exp Med Biol 2017; 975(1): 17–25. doi: 10.1007/ 978-94-024-1079-2_2.
39. Lee E, Lee J, Kim E. Excitation/ inhibition imbalance in animal models of autism spectrum disorders. Biol Psychiatry 2017; 81(10): 838–847. doi: 10.1016/ j.biopsych.2016.05.011.
40. Silverman JL, Smith DG, Rizzo SJ et al. Negative allosteric modulation of the mGluR5 receptor reduces repetitive behaviors and rescues social deficits in mouse models of autism. Sci Transl Med 2012; 4(131): 131ra51. doi: 10.1126/ scitranslmed.3003501.
41. Silverman JL, Oliver CF, Karras MN et al. AMPAKINE enhancement and social interaction in the BTBR mouse model of autism. Neuropharmacology 2013; 64(1): 268–282. doi: 10.1016/ j.neuropharm.2012.07.013.
42. Inoue K, Furukawa T, Kumada T et al. Taurine inhibits K+–Cl– cotransporter KCC2 to regulate embryonic Cl– homeostasis via with-no-lysine (WNK) protein kinase signaling pathway. J Biol Chem 2012; 287(25): 20839–20850. doi: 10.1074/ jbc.M111.319418.
43. Finegold SM, Molitoris D, Song Y et al. Gastrointestinal microflora studies in late-onset autism. Clin Infect Dis 2002; 35 (Suppl 1): S6–S16. doi: 10.1086/ 341914.
44. Sandler RH, Finegold SM, Bolte ER et al. Short-term benefit from oral vancomycin treatment of regressive--onset autism. J Child Neurol 2000; 15(7): 429–435. doi: 10.1177/ 088307380001500701.
45. Chini B, Leonzino M, Braida D et al. Learning about oxytocin: pharmacologic and behavioral issues. Biol Psychiatry 2014; 76(5): 360–366. doi: 10.1016/ j.biopsych.2013.08.029.
46. Erdman SE, Poutahidis T. Probiotic „glow of health“: it is more than skin deep. Benef Microbes 2014; 5(2): 109–119. doi: 10.3920/ BM2013.0042.
47. Sanctuary MR, Kain JN, Chen SY et al. Pilot study of probiotic/ colostrum supplementation on gut function in children with autism and gastrointestinal symptoms. PLoS One 2019; 14(1): e0210064. doi: 10.1371/ journal.pone.0210064.
48. Grimaldi R, Gibson GR, Vulevic J et al. A prebiotic intervention study in children with autism spectrum disorders (ASDs). Microbiome 2018; 6(1): 133. doi: 10.1186/ s40168-018-0523-3.
49. Arnold LE, Luna RA, Williams K et al. Probiotics for gastrointestinal symptoms and quality of life in autism: a placebo-controlled pilot trial. J Child Adolesc Psychopharmacol 2019; 29(9): 659–669. doi: 10.1089/ cap.2018.0156.
50. Inoue R, Sakaue Y, Kawada Y et al. Dietary supplementation with partially hydrolyzed guar gum helps improve constipation and gut dysbiosis symptoms and behavioral irritability in children with autism spectrum disorder. J Clin Biochem Nutr 2019; 64(3): 217–223. doi: 10.3164/ jcbn.18-105.
51. Liu J, Liu X, Xiong XQ et al. Effect of vitamin A supplementation on gut microbiota in children with autism spectrum disorders – a pilot study. BMC Microbiol 2017; 17(1): 204. doi: 10.1186/ s12866-017-1096-1.
52. Bagdasarian N, Rao K, Malani PN. Diagnosis and treatment of Clostridium difficile in adults: a systematic review. JAMA 2015; 313(4): 398–408. doi: 10.1001/ jama.2014.17103.
53. Moayyedi P, Surette MG, Kim PT et al. Fecal microbiota transplantation induces remission in patients with active ulcerative colitis in a randomized controlled trial. Gastroenterology 2015; 149(1): 102–109. doi: 10.1053/ j.gastro.2015.04.001.
54. Vrieze A, Van Nood E, Holleman F et al. Transfer of intestinal microbiota from lean donors increases insulin sensitivity in individuals with metabolic syndrome. Gastroenterology 2012; 143(4): 913–916. doi: 10.1053/ j.gastro.2012.06.031.
55. Kang DW, Adams JB, Gregory AC et al. Microbiota transfer therapy alters gut ecosystem and improves gastrointestinal and autism symptoms: an open-label study. Microbiome 2017; 5(1): 10. doi: 10.1186/ s40168-016-0225-7.
56. Kang DW, Adams J, Coleman D et al. Long-term benefit of microbiota transfer therapy on autism symptoms and gut microbiota. Sci Rep 2019; 9(1): 5821. doi: 10.1038/ s41598-019-42183-0.
57. Zhao H, Gao X, Xi L et al. Mo1667 Fecal microbiota transplantation for children with autism spectrum disorder. Gastrointest Endosc 2019; 89(Suppl): AB512–AB513. doi: 10.1016/ j.gie.2019.03.857.
Štítky
Detská neurológia Neurochirurgia NeurológiaČlánok vyšiel v časopise
Česká a slovenská neurologie a neurochirurgie
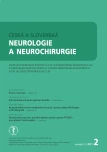
2021 Číslo 2
- Metamizol jako analgetikum první volby: kdy, pro koho, jak a proč?
- Fixní kombinace paracetamol/kodein nabízí synergické analgetické účinky
- Kombinace metamizol/paracetamol v léčbě pooperační bolesti u zákroků v rámci jednodenní chirurgie
- Tramadol a paracetamol v tlumení poextrakční bolesti
- Kombinace paracetamolu s kodeinem snižuje pooperační bolest i potřebu záchranné medikace
Najčítanejšie v tomto čísle
- Morton’s neuralgia, metatarsalgia
- Moyamoya disease
- Correct and incorrect naming of pictures for the more demanding written Picture Naming and Immediate Recall test (door PICNIR)
- Gut microbiota and autism spectrum disorders