Current use of the automatic retinal oximetry. Review
Authors:
P. Hübnerová 1,2; P. Mlčák 1,2; I. Šínová 1; M. Karhanová 1; M. Šín 1,3
Authors place of work:
Oční klinika Lékařské fakulty Univerzity Palackého v Olomouci
a Fakultní nemocnice Olomouc, přednostka: MUDr. Klára Marešová, Ph. D., FEBO
1; Ústav fyziologie Lékařské fakulty Univerzity Palackého
v Olomouci, přednosta: doc. MUDr. Jiří Nečas, CSc.
2; Oční klinika 1. lékařské fakulty Univerzity Karlovy a Ústřední vojenské nemocnice Praha, přednosta: doc. MUDr. Martin Šín, Ph. D., FEBO
3
Published in the journal:
Čes. a slov. Oftal., 76, 2020, No. 1, p. 3-11
Category:
doi:
https://doi.org/10.31348/2020/1
Summary
Purpose: To inform about possible use of the automatic retinal oximetry for the retinal oxygen saturation measurement in the eye and systemic diseases.
Methods: We performed a literature review dealing with issues of retinal oxygen saturation monitoring by dual non-invasive retinal oximetry Oxymap T1 (Oxymap ehf. Reykjavík, Iceland).
Results: We have found two main strains writing our paper on retinal oxygen saturation eye diseases. The first section concerns diseases created by having hypoxia as its main pathological factor - for example diabetes mellitus and retinal vein occlusion. The second group deals with atrophy as the main pathological mechanism which is typical for decreasing retinal oxygen consumption – for example glaucoma or retinitis pigmentosa (the second one named is not included in our work). Oximetry in systemic diseases creates a relatively new chapter of this branch with a very big potential of interdisciplinary cooperation for the future. It is possible the cooperation will not only include diabetologists but also neurologists (for example, in diseases like sclerosis multiplex or Devic‘s, Alzheimer‘s and Parkinson‘s disease) and haematologists (retinal oxygen saturation changes in patients with different rheological attributes of blood).
Conclusion: Retinal oxygen saturation measuring by automatic retinal oximetry is a relatively new method with scientifically confirmed high reproducibility of results. Currently it is the only experimental method with vast potentials not only in the realm of the possibility of observing eye diseases (diabetic retinopathy, retinal vein occlusion or glaucoma) but also in developing interdisciplinary cooperation with diabetologists, neurologists and haematologists.
Keywords:
automatic retinal oximetry – retinal oxygen saturation – Diabetic retinopathy – Multiple sclerosis – glaukom
INTRODUCTION
The aim of the study is to familiarise readers with the possible use of automatic retinal oximetry for the measurement of retinal oxygen saturation in ocular and general pathologies. Automatic retinal oximetry is a relatively new method, with scientifically confirmed high reproducibility of results. To date only one instrument is available in the Czech Republic, at the Department of Ophthalmology of Olomouc University Hospital.
The largest studies dealing with the monitoring of retinal oxygen parameters in ocular pathologies focus on diabetic retinopathy or retinal vein occlusion, less widely represented are glaucoma or retinitis pigmentosa (the last of these is not included in our analysis). Oximetry in general pathologies forms a relatively new chapter in this branch, with large potential for interdisciplinary co-operation in future. Already commenced co-operation with diabetologists could be supplemented also by co-operation with neurologists focusing on understanding the pathophysiology of diseases such as multiple sclerosis, Devic’s, Alzheimer’s or Parkinson’s diseases. Last but not least, we would also like to mention incipient co-operation with haematologists, focusing on changes of retinal oxygen saturation in patients with altered blood rheological properties.
Methods of measuring oxygen saturation in the retina and in the vitreous body – history
For many years, measurement of the level of oxygen in the retina and vitreous body was limited as a result of the need for invasive methods of measurement [6,59]. Invasive measurement, the greatest limitation of which was the necessity to penetrate the surface of the eye, was first used in the 1980s. Polarographic electrodes sensitive to oxygen and probes which contained colouring sensitive to oxygen were used. These invasive probes were inserted into the vitreous cavity in order to measure partial oxygen pressure, for example above the retina or above the optic nerve (most often during an intraocular operation). It is assumed that the pre-retinal value of partial oxygen pressure has a similar value as its partial pressure in the inner retina [2,47].
Non-invasive blood oximetry was first performed in Germany in 1930, and the first ear oximeter was produced in 1936 [44]. Hickam et al. [28] were the first to attempt to use a similar principle on eyes. They used special filters in order to obtain images of the ocular fundus with two different wavelengths of light. Through subsequent densitometric measurement of the photographic films they estimated the light intensities, which were then used to calculate retinal oxygen saturation of the blood vessel in a certain section. In these pioneering studies they also succeeded in demonstrating changes in retinal oxygen saturation depending on the concentration of oxygen in inhaled air [28].
A great advance in research in the field of oximetry using two wavelengths came at the end of the 20th century, when Beach et al. [7] and Tiedeman et al. [52] used two wavelengths for the calculation of oximetry, similarly as performed many decades previously by Hickam et al [28]. The main improvement was achieved thanks to the use of digital camera technology, which had not previously been used in oximetry.
Beach et al. [7] also attempted to develop the principles that led to a correction of the influence of the diameter of the blood vessel and different pigmentation of the ocular fundus. The image from the retinal camera was divided so that two images of the same area of the retina were obtained at the same time. One image had a wavelength sensitive to oxygen saturation and the second image was non-sensitive to oxygen saturation. Both of these images were obtained at the same time by a single flash on the same camera sensor, thereby eliminating the problem of eye movement. These studies laid the foundation for the development of instruments for non-invasive measurement of oxygen saturation in retinal blood vessels [7].
Methods of measuring retinal oxygen saturation – present situation
From the above it is evident that with the arrival of the non-invasive automatic retinal oximeter, a watershed was reached in research into retinal oxygen parameters. The dual non-invasive retinal oximeter Oxymap T1 (Oxymap ehf., Reykjavik, Iceland) is composed of two digital cameras, a special optical adapter, an image divider and two narrow band filters. The entire device is attached to a standard retinal camera (TRC-50 DX, Topcon corp., Tokyo, Japan), which is used to obtain two images of the retina with different wavelengths in a single moment (Fig. 1) [23,24,25]. Light absorbance of the blood vessels is influenced by the absorbance (colour) of the blood in the vessel, which is determined by the presence of oxygenated or non-oxygenated haemoglobin A. Light absorbance of oxygenated and non-oxygenated haemoglobin A differs in several wavelengths, but in some it is the same. These wavelengths are referred to as isosbestic, and one of these is the wavelength of 570 nm. Among others, the wavelength of 600 nm (Graph 1) is non-isosbestic. In comparison with the fluctuating colour of the blood vessels, the colour of the retinal fundus is relatively constant, and is not excessively influenced by oxygen saturation. In this manner it is possible to describe the optical density of the blood vessel. The optical density of the blood vessel is heavily influenced by oxygen saturation upon the production of an image by light with a wavelength of 600 nm (the arteries are markedly darker than the veins, Fig. 2), but not at the referential wavelength of 570 nm (arteries and veins have a virtually identical appearance, Fig. 3). The ratio of optical densities at wavelengths of 600 nm and 570 nm gives the “optical density ratio”, which is inversely and approximately linearly dependent on oxygen saturation [23,24,25].
The results obtained by measurement on healthy patients were used for calibration of the Oxymap T1 instrument. These values were compared with measurements on an already calibrated instrument [43]. Special software (Oxymap analyser software 2.2.1, version 3847; Oxymap ehf.) measures the luminosity of selected points on the obtained images at the aforementioned wavelengths. The measurement points are placed by the software onto large blood vessels and the retinal fundus along the blood vessels, and the software allocates an oxygen saturation value to each point on the blood vessel, expressed with the aid of a colour scale (Fig. 4).
Retinal oximetry in ocular pathologies
The largest studies focusing on monitoring oxygen parameters on the retina in ocular pathologies, in which it is possible to demonstrate a large reproducibility of results, can be divided into two main categories. The first group comprises pathologies in which hypoxia (ischemia) is the main pathophysiological factor. The main representatives of this group are diabetic retinopathy and retinal vein occlusion. In the second group of pathologies the main pathophysiological mechanism is atrophy, which leads to a reduction of retinal oxygen consumption. Examples of diseases in this group are glaucoma and retinitis pigmentosa (studies examining retinitis pigmentosa are not included in this analysis).
Diabetic retinopathy
Diabetic retinopathy (DR) is a typical late microvascular complication of diabetes, which progressively develops with varying degrees of severity in the majority of patients with diabetes [19,58]. It is characterised by damage to the retinal capillaries, thickening of the basal membranes, obliteration or distension of capillaries with the formation of microaneurysms, and subsequent poor blood distribution in the retina [11,33,34,41]. The final result is areas of hypoxia and ischemia, which lead to the development of proliferative diabetic retinopathy (PDR), or the development of diabetic macular edema (DME) [33].
The precise biochemical processes in connection with increased glycaemia are complex and as yet not entirely known. In this area the development of automatic retinal oximetry has provided an ideal research tool. To date 6 cross-sectional studies have been published, describing the dependency of changes of oxygen saturation in retinal blood vessels upon a background of DR [22,25,30,31,32,49].
In all 6 studies higher venous oxygen saturation (vSatO2) was described in patients with proliferative DR in comparison with a control group, and at the same time in 5 studies higher arterial oxygen saturation (aSatO2) was described. The changes of oxygen saturation in diabetics were contributed to by a minimum of three known mechanisms: 1. capillary non-perfusion and shunting of certain areas of the retina, 2. thickening of the capillary walls and 3. higher affinity of haemoglobin for oxygen in diabetics.
It has been demonstrated that in diabetic patients an occlusion of certain capillaries takes place, while others are dilated. In patients with diabetic retinopathy we demonstrate non-perfusion and dilation of retinal capillaries and arterioles in different areas of the retina by means of fluorescence angiography. The result of these changes is that a large amount of blood flows through the dilated capillaries at a high speed from the arteries to the veins, without a sufficient quantity of oxygen reaching the target tissue (retina). This mechanism leads to an increase of saturation in the venous blood [11,34].
A further mechanism which could contribute to changes in the resulting oxygen saturation of the retinal blood vessels is thickening of the capillary walls in diabetics, which has been unequivocally demonstrated [5,41]. This thickening is responsible for impairing the diffusion of oxygen from the blood into the retinal tissue, and thereby contributes to a greater quantity of oxygen in the venous blood.
A third mechanism which may contribute to an overall increase of saturation in the retinal blood vessels is an increase of the affinity of haemoglobin for oxygen together with an increase of haematocrit in patients with diabetes [18,36]. The higher affinity of haemoglobin for oxygen and the larger number of red blood cells could explain the higher values of saturation in the arterial and venous channel.
The precise determination of the proportion of the individual mechanisms on resulting retinal saturation is thus far unclear. At the same time, it is probable that further, as yet unknown factors exist, which contribute to changes in oxygen saturation in the retinal channel. In future it shall be necessary to conduct further studies in order to gain a deeper understanding of all the mechanisms.
The great majority of studies published to date are cross-sectional studies. This represents a significant disadvantage, because even though a pronounced correlation was found in all the cases published to date between the degree of affliction and the values of retinal saturation (mostly an increase of saturation of venous blood), it is not possible to use these values for clinical decision-making due to the low specificity of this value (certain saturation values are common for all stages of DR). Up to now a morphological approach evaluating changes on the retina of individual patients has been used for indication of commencement of therapy (laser, pars plana vitrectomy, anti-VEGF therapy). It is therefore possible to discuss the future possibility of more precise individual monitoring of patients over time, in which fluctuating values of oxygen saturation will be a sensitive indicator for the commencement of therapy. It ensues from all the studies dealing with oxygen saturation published to date that this value represents a more sensitive indicator, and could become one of the criteria for the commencement of timely therapy. If therapy has already been commenced (e.g. laser or anti-VEGF), a further large possibility opens up for the use of retinal oximetry for the measurement of the effectiveness of this therapy, as well as the titration of a quantity of applied laser points. To date no objective method of measurement exists which could evaluate the effectiveness of laser therapy and reduce the possibility of this irreversible process on the retina. We are still at the beginning of the path toward the effective use of retinal oximetry in diabetic patients, and studies with a longer observation period represent a very important step, which could confirm these assumptions and aid the introduction of this approach into clinical practice.
Retinal vein occlusion
Venous occlusion is a significant cause of loss of sight among the older population worldwide. Among retinal vascular pathologies it is the second most common cause of loss of sight – immediately behind diabetic retinopathy [13]. We can divide venous occlusion into two main types. Branch Retinal Vein Occlusion (BRVO), which occurs upon occlusion of the peripheral part of the venous vascular channel and is usually caused by compression of the venous branch by a sclerotic retinal arteriole in the place of arteriovenous crossing. The second type is Central Retinal Vein Occlusion (CRVO), which occurs upon occlusion of the central retinal vein in the region of the lamina cribrosa. In both cases, a halting and turbulence of blood flow occurs at critical points, thereby creating the conditions for the formation of a thrombus.
A thrombus causes a further significant retardation of the blood flow, either throughout the entire retina (CRVO) or only in occluded areas, depending on the scope of the affliction (BRVO). In the case of central occlusion (CRVO), however, in most cases a complete halting of blood flow does not take place, even in the case of complete blockage by a thrombus [27]. This flow is enabled by the presence of tributaries of the central retinal vein localised anteriorly from the point of occlusion (most often in the region of the lamina cribrosa), which supplement the collateral circulation [51]. Retardation of the blood flow upon a background of central retinal vein occlusion reduces the supply of oxygen to the area of the inner retina and leads to hypoxia [59,60]. Studies have demonstrated a reduction of the speed of the through-flow of blood in the retinal veins with the aid of Doppler flowmetry [53,54], a reduction of the speed of blood flow in the capillaries with the aid of a Heidelberg retinal flowmeter [4], and an extension of the time of arteriovenous passage upon fluorescence and indocyanine angiography [3,38,39]. The reduction of blood through-flow leads to hypoxia [23,59,60], and subsequently to an increase in the production of VEGF [37,40]. The result is vasodilation and hyperfusion, which potentiates the vicious circle, worsening haemorrhage and edema. In addition to this, exudation into the retinal extracellular spaces, which have a limited capacity, may lead to an increase in interstitial flow and a further reduction of the through-flow of blood. In future the successful use of automatic retinal oximetry is envisaged in clinical practice for retinal vein occlusion.
The results of measurement of patients with CRVO are very promising. Although only isolated studies have been conducted to date, CRVO is considered the diagnosis with the greatest potential for the use of automatic retinal oximetry. So far 5 pilot cross-sectional studies have been published focusing on automatic retinal oximetry in retinal vein occlusions. In three cases the subject of the study was CRVO, and in two BRVO.
The results of retinal oximetry in patients with CRVO appear to be more consistent than in patients with diabetes. All three studies demonstrated a reduction in vSatO2 in eyes with CRVO [16,23,55]. By contrast, aSatO2 was not changed in any of these studies upon a comparison of the afflicted eyes with healthy controls. A reduction of vSatO2 is a reflection of the amount of oxygen which remains in the venous system following flow through the retinal micro-circulation. Based on speculations by Eliasdottir et al., it is possible to assume that a decrease of saturation is the result of a reduction of through-flow of blood or an increase of oxygen extraction. Occlusion has an influence on the through-flow of blood in blood vessels, and thereby reduces the supply of oxygen to the tissues. Hypoxic tissues extract more oxygen from the reduced quantity of blood flowing through the blood vessel, and thereby cause a decrease of the oxygen level in the retinal veins [16]. Campochiaro et al. determined that the application of ranibizumab reduces the progression of capillary non-perfusion of the retina in CRVO. With the reduction of capillary non-perfusion, the through-flow of blood in the retina is increased, leading to an increase of vSatO2 [9]. This finding is in accordance with the finding of Traustason et al., who confirmed an increase of vSatO2 in patients with CRVO following therapy with anti-VEGF preparations [55].
In patients with BRVO the situation is somewhat different. In both studies the results were markedly heterogeneous and inconsistent [24,35], which is however linked to the greater variability of the severity and various symptoms with regard to the scope of occlusion. The heterogeneity of the results is also contributed to by the practical impossibility of standardising the analysis of the results. A significant contribution to this issue has been brought by the latest study by Šínová et al., in which a correlation was observed between the results of oxygen saturation and the ischemic index of retinal occlusion [50]. The ischemic index (ISI) of venous occlusion is a relatively new concept, which is the product of the introduction of wide-angle imaging systems into clinical practice, and was first mentioned in the study by Tsui et al [57]. Wide-angle systems enable imaging of the retina within a range of as much as 200°, in comparison with the standard imaging within the range of 35°-50°. Thanks to this imaging of the remote periphery, we can determine the precise ratio of the perfused and non-perfused retina. This ratio, which takes on values of 0-1, is referred to as the ischemic index. The results of the study by Šínová et al. are fully in accordance with the previous studies, vSatO2 is significantly lower in cases of CRVO and BRVO. In addition, a statistically significant negative correlation has been observed between ISI and venous saturation in the group of CRVO. A significant positive correlation was determined between ISI and the arteriovenous difference (A-V difference) in the group of CRVO. ISI represents a quantitative parameter, expressing the degree of ischemia (severity) of retinal vein occlusion (RVO) [50]. Upon an increase in ISI, there is an increase in the risk of macular edema and neovascularisation [46,57]. The use of automatic retinal oximetry also represents a quantitative evaluation of RVO. The results indicate that the severity of CRVO may be expressed either by ISI or by the results of retinal oximetry. Also in accordance with the above are the results of diabetic patients, in whom a relationship was also found between the values of ISI and the values of oxygen saturation in the retina [21].
Thanks to the results published so far, determining a correlation between ISI parameters and the values of retinal oxygen saturation, it is possible to expect that the general ischemic condition of the retina could be observed with the aid of retinal oximetry. On the other hand, this does not apply in the case of BRVO, in which there was no such similar correlation in accordance with the other studies.
GLAUCOMA
Glaucoma is an ocular pathology, considered to be an optic neuropathy combined with apoptosis of the retinal ganglion cells and loss of the visual field [17]. The main risk factor of the onset and progression of glaucoma is increased intraocular pressure, but even upon a reduction of pressure the pathology may progress [8]. There is an ever increasing amount of evidence that the through-flow of blood in eyes with glaucoma is reduced, or that the regulation of blood through-flow is impaired in comparison with eyes without glaucoma [20]. However, it is not clear as to whether these changes are primary or secondary, thus whether they are the cause or the effect of glaucomatous atrophy of the retinal ganglion cells and the optic nerve.
In all the studies published to date, oxygen saturation in the retinal arteries was relatively stable and was not influenced by the degree of severity of glaucoma. However, oxygen saturation in retinal veins increased in all the studies upon a background of progression of glaucoma. This increase in saturation corresponds both with functional change (size of defect in visual field) and with morphological parameters (thickness of retinal nerve fibre layer – RNFL – and surface of the neuroretinal rim). The A-V difference is logically in a mirrored dependency with regard to both of the aforementioned parameters as the value of venous saturation. A lower A-V difference, together with a reduction of ocular through-flow of blood in the case of glaucoma, as can be seen in previous studies, indicates a lower consumption of oxygen in the retina in dependency upon a reduction of the quantity and function of the nerve cells in the retina [1,26,42]. In the case of glaucoma, evaluation of the cause and effect of this phenomenon is highly complex, and it can be stated that on the basis of our current knowledge it is impossible to provide an answer to this question. From this perspective, interesting data has been presented by the recent Leuven Eye Study, which demonstrated a difference in the values of oxygen saturation in patients with open-angle glaucoma and normotensive glaucoma [1]. This data indicates that intraocular pressure need not be the sole factor in the pathogenesis of glaucoma, and as was already previously assumed, at least in the case of normotensive glaucoma the proportion of the vascular component is more significant. A very significant obstacle to answering the question of the issue of vascular changes in the pathogenesis of glaucoma with the aid of using automatic retinal oximetry is presented by the absence of a longer observation time of measurement, with regard to the fact that vascular changes may occur intermittently, similarly to changes of intraocular pressure, which may increase during the night [10]. The resulting values of oxygen saturation could also have been altered by the influence of anti-glaucomatous agents, as was described in the study conducted by S. Traustason et al. [56]. All these types of disadvantages emphasise the influence of an increase of venous oxygen saturation (and a mirrored view of the A-V difference) as a secondary effect of cell atrophy, although the reality (at least in the case of normotensive glaucomas) may be different.
In the case of glaucoma, the question of cause and effect, as has been outlined in the previous text about vascular changes, represents the chief scientific problem at present, which it will be necessary to resolve in the forthcoming period. From a clinical perspective, automatic retinal oximetry could become an appropriate supplement to current morphological imaging methods (OCT – optical coherence tomography and HRT – Heidelberg retinal tomography) in the diagnosis and monitoring of the effectiveness of glaucoma treatment.
Retinal oximetry and changes of oxygen saturation in general pathologies
Oximetry in general pathologies represents a relatively new chapter in this branch, with large potential for future interdisciplinary co-operation. Routine co-operation with diabetologists is now being followed by co-operation with neurologists, focusing on understanding the pathophysiology for example of pathologies of the type of multiple sclerosis and Devic’s, Alzheimer’s and Parkinson’s diseases. Last but not least, we would also like to mention the incipient co-operation with haematologists, focusing on changes in oxygen saturation in patients with altered blood rheological properties.
Automatic retinal oximetry and neurological diseases
The retina, formed as an exagination of the neuroectoderm, is an ideal structure for assessing the condition of nerve structures, because there is an absence of myelin here, and the ganglion cells are directly accessible for observation [29]. Studies with optical coherence tomography have demonstrated a weakening of the nerve fibre layer and other structural anomalies in the retina in the case of brain disorders such as Alzheimer’s disease (AD), multiple sclerosis (MS) and Parkinson’s disease [12]. Retinal oximetry offers an opportunity to supplement morphological imaging, on the assumption that metabolic changes of brain disorders may be manifested on the retina. The actual potential of this examination in this area shall be demonstrated in the forthcoming years.
Einarsdottir et al. were the first to demonstrate metabolic changes in the retina upon a background of brain disorders, in which they observed oximetric abnormalities in the retina of patients with mild to medium-severe dementia upon a background of AD in comparison with a healthy cohort. The values of retinal oxygen saturation in the arterioles and venules in patients with medium-severe AD were significantly higher in comparison with healthy individuals [14].
Einarsdottir et al. also conducted a pilot measurement of eight patients with MS and a previous medical history of neuritis of the optic nerve. Venous oxygen saturation was significantly increased in patients with MS in comparison with healthy individuals. The arteriovenous difference in patients with MS was lower than in healthy individuals. No difference in oxygen saturation in arterioles was recorded between patients with MS and healthy individuals [15].
In both of the aforementioned studies, which focused on the chronic stage of the pathology, there was an increase of vSatO2, which corresponds with the degree of atrophy, and thereby with reduced oxygen consumption. We can observe a similar pattern in atrophic pathologies of the retina, such as retinitis pigmentosa or glaucoma [1,61].
Interesting data in connection with the above was produced by a recent study conducted by our team in this area, which focused on the acute stage of neuritis of the optic nerve upon a background of MS. The arteriovenous difference was increased in the affected eye of patients in comparison with the other, unaffected eye. This study demonstrates that changes in the optic nerve lead to an increase of oxygen consumption on the retina. The presumed explanation of this phenomenon is that the RNFL is composed of axons of ganglion cells that may be influenced by axonal inflammation, which may then lead to increased oxygen consumption upon higher metabolic requirements of the ganglion cells affected by immune processes [48]. This mechanism has been described in experimental neuritis of the optic nerve in mice [45].
Haematological diseases
In addition to neurologists and diabetologists, the results of retinal oximetry may also be of interest to haematologists. In connection with the composition of blood and changes of blood rheological properties, up to this day one prospective study originating from Denmark has been published, focusing among other factors on the oxygen parameters on the retina measured by retinal oximetry in a small sample of patients with myeloproliferative neoplasms (chronic myeloid leukaemia, polycythemia vera, essential thrombocythemia). In this study, Willerslev et al. describe changes following cytoreduction therapy in the sense of an increase of arterial saturation [61].
We see the sense of co-operation with haematologists above all in the potential evaluation of retinal changes as an image of the state of the entire blood micro-circulation, thus as a prediction of circulation complications in patients with altered blood rheology. However, more extensive studies are required for a relevant evaluation.
CONCLUSION
Measurement of oxygen saturation with the aid of automatic retinal oximetry is a relatively new method, with scientifically confirmed high reproducibility of results. At present it represents an experimental method, but we regard it as having large future potential, not only in the possibility of observing ocular pathologies, but especially in the observation of retinal oxygen parameters in connection with general pathologies. The developing interdisciplinary co-operation with diabetologists, neurologists and haematologists attests to the broad field of research possibilities. It is not yet possible at present to answer with certainty the question as to whether this method will be usable also for clinical practice.
The authors of the study declare that no conflict of interest exists in the compilation, theme and subsequent publication of this professional communication, and that it is not supported by any pharmaceuticals company. The authors further declare that the study has not been submitted to any other journal or printed elsewhere.
Zdroje
1. Abegão Pinto, L., Willekens, K., Van Keer, K. et al.: Ocular blood flow in glaucoma–the Leuven Eye Study. Acta Ophthalmol, 94(6); 2016: 592-598.
2. Alder, VA., Cringle, SJ.: Vitreal and retinal oxygenation. Graefes Arch Clin Exp Ophthalmol, 228; 1990: 151–157.
3. Arsene, S., Giraudeau, B., Le Lez, ML. et al.: Follow up by colour Doppler imaging of 102 patients with retinal vein occlusion over 1 year. Br J Ophthalmol, 86; 2002: 1243–1247.
4. Arvas, S., Ocakoglu, O., Ozkan, S.: The capillary blood flow in ischaemic type central retinal vein occlusion: the effect of laser photocoagulation. Acta Ophthalmol Scand, 80; 2002: 490–494.
5. Ashton, N.: Vascular basement membrane changes in diabetic retinopathy. Montgomery lecture 1973. Br J Ophthalmol, 58; 1974: 344–366.
6. Barbazetto, IA., Liang, J., Chang, S. et al.: Oxygen tension in the rabbit lens and vitreous befor and after vitrectomy. Exp Eye Res, 78(5); 2004: 917-924.
7. Beach, JM., Schwenzer, KJ., Srinivas, S. et al.: Oximetry of retinal vessels by dual-wavelength imaging: calibration and influence of pigmentation. J Appl Physiol, 86; 1999: 748–758.
8. Broadway, DC., Drance, SM.: Glaucoma and vasospasm. Br J Ophthalmol, 82; 1998: 862–870.
9. Campochiaro, PA., Bhisitkul, RB., Shapiro, H. et al.: Vascular endothelial growth factor promotes progressive retinal nonperfusion in patients with retinal vein occlusion. Ophthalmology, 120(4); 2013: 795-802.
10. Cheng, J., Kong, X., Xiao, M. et al.: Twenty four hour pattern of intraocular pressure in untreated patients with primary open angle glaucoma. Acta Ophthalmol, 94(6); 2016: 460-467.
11. Cogan, DG., Kuwabara, T.: Capillary Shunts in the Pathogenesis of Diabetic Retinopathy. Diabetes, 12; 1963: 293–300.
12. Costello, F.: Evaluating the use of optical coherence tomography in optic neuritis. Mult Scler Int, Volume 2011: doi:10.1155/2011/148394
13. Cugati, S., Wang, JJ., Rochtchina, E. et al.: Ten-year incidence of retinal vein occlusion in an older population: the Blue Mountains Eye Study. Arch Ophthalmol, 124(5); 2006: 726-732.
14. Einarsdottir, AB., Hardarson, SH., Kristjansdottir, JV. et al.: Retinal oximetry imaging in Alzheimer’s disease. J Alzheimers Dis, 49(1); 2015: 79-83.
15. Einarsdottir, AB., Olafsdottir, OB., Hjaltason, H. et al.: Retinal oximetry is affected in multiple sclerosis. Acta Ophthalmol, 96(5); 2018: 528-530.
16. Eliasdottir, TS., Bragason, D., Hardarson, SH. et al.: Venous oxygen saturation is reduced and variable in central retinal vein occlusion. Graefes Arch Clin Exp Ophthalmol, 253; 2015: 1653–1661.
17. Foster, PJ., Buhrmann, R., Quigley, HA. et al.: The definition and classification of glaucoma in prevalence surveys. Br J Ophthalmol, 86; 2002: 238–242.
18. Graham, JJ., Ryall, RG., Wise, PH.: Glycosylated haemoglobin and relative polycythaemia in diabetes mellitus. Diabetologia, 18(3); 1980: 205-207.
19. Grauslund, J., Green, A., Sjolie, AK.: Prevalence and 25 year incidence of proliferative retinopathy among Danish type 1 diabetic patients. Diabetologia, 52; 2009: 1829-1835.
20. Grieshaber, MC., Flammer, J.: Blood flow in glaucoma. Curr Opin Ophthalmol, 16; 2005: 79-83.
21. Guduru, A., Martz, TG., Waters, A. et al.: Oxygen Saturation of Retinal Vessels in All Stages of Diabetic Retinopathy and Correlation to Ultra-Wide Field Fluorescein Angiography. Invest Ophthalmol Vis Sci, 57(13); 2016: 5278-5284.
22. Hammer, M., Vilser, W., Riemer, T. et al.: Diabetic patients with retinopathy show increased retinal venous oxygen saturation. Graefes Arch Clin Exp Ophthalmol, 247(8); 2009: 1025-1030
23. Hardarson, SH., Stefansson, E.: Oxygen saturation in central retinal vein occlusion. Am J Ophthalmol, 150; 2010: 871–875.
24. Hardarson, SH., Stefansson, E.: Oxygen saturation in branch retinal vein occlusion. Acta Ophthalmol, 90; 2012a: 466–470.
25. Hardarson, SH., Stefansson, E.: Retinal oxygen saturation is altered in diabetic retinopathy. Br J Ophthalmol, 96; 2012b: 560–563.
26. Harris, A., Sergott, RC., Spaeth, GL. et al.: Color Doppler analysis of ocular vessel blood velocity in normaltension glaucoma. Am J Ophthalmol, 118; 1994: 642–649.
27. Hayreh, SS.: Prevalent misconceptions about acute retinal vascular occlusive disorders. Prog Retin Eye Res, 24; 2005: 493–519.
28. Hickam, JB., Sieker, HO., Frayser, R.: Studies of retinal circulation and A-V oxygen difference in man. Trans Am Clin Climatol Assoc, 71; 1959: 34–44.
29. Hu, SJ., You, YA., Zhang, Y.: A study of retinal parameters measured by optical coherence tomography in patients with multiple sclerosis). Int J Ophthalmol, 8(6); 2015: 1211-4.
30. Jørgensen, CM., Bek, T.: Increasing oxygen saturation in larger retinal vessels after photocoagulation for diabetic retinopathy. Invest Ophthalmol Vis Sci, 55(8); 2014: 5365-5369.
31. Jørgensen, CM., Hardarson, SH., Bek, T.: The oxygen saturation in retinal vessels from diabetic patients depends on the severity and type of vision-threatening retinopathy. Acta Ophthalmol, 92(1); 2014: 34-39.
32. Khoobehi, B., Firn, K., Thompson, H. et al.: Retinal arterial and venous oxygen saturation is altered in diabetic patients. Invest Ophthalmol Vis Sci, 54; 2013: 7103–7106.
33. Kohner, EM.: Diabetic retinopathy. BMJ, 307; 1993: 1195–1199.
34. Kuwabara, T., Cogan, DG.: Retinal vascular patterns. VI. Mural cells of the retinal capillaries. Arch Ophthalmol., 69; 1963: 492–502.
35. Lin, LL., Dong, YM., Zong, Y. et al.: Study of retinal vessel oxygen saturation in ischemic and non-ischemic branch retinal vein occlusion. Int J Oph, 9(1); 2016: 99.
36. Linsenmeier, RA., Braun, RD., McRipley, MA. et al.: Retinal hypoxia in long-term diabetic cats. Invest Ophthalmol Vis Sci, 39(9); 1998: 1647-57.
37. Noma, H., Funatsu, H., Mimura, T. et al.: Vitreous levels of pigment epithelium-derived factor and vascular endothelial growth factor in macular edema with central retinal vein occlusion. Curr Eye Res, 36; 2011: 256–63.
38. Paques, M., Baillart, O., Genevois, O. et al.: Systolodiastolic variations of blood flow during central retinal vein occlusion: exploration by dynamic angiography. Br J Ophthalmol, 89; 2005: 1036–40.
39. Paques, M., Garmyn, V., Catier, A. et al.: Analysis of retinal and choroidal circulation during central retinal vein occlusion using indocyanine green videoangiography. Arch Ophthalmol, 119; 2001: 1781–7.
40. Pe’er, J., Folberg, R., Itin, A. et al.: Vascular endothelial growth factor upregulation in human central retinal vein occlusion. Ophthalmology, 105; 1998: 412–16.
41. Roy, S., Ha, J., Trudeau, K. et al.: Vascular Basement Membrane Thickening in Diabetic Retinopathy. Curr Eye Res, 35; 2010: 1045–1056.
42. Rojanapongpun, P., Drance, SM., Morrison, BJ.: Ophthalmic artery flow velocity in glaucomatous and normal subjects. Br J Ophthalmol, 77; 1993: 25–29.
43. Schweitzer, D., Leistritz, L., Hammer, M. et al.: Calibration- free measurement of the oxygen saturation in human retinal vessels. Ophthalmic Technologies V., Proc SPIE 2393; 1995: 210–218.
44. Severinghaus, JW.: Monitoring oxygenation. J Clin Monit Comput, 25; 2011: 155–161.
45. Shindler, KS., Ventura, E., Dutt, M. et al.: Inflammatory demyelination induces axonal injury and retinal ganglion cell apoptosis in experimental optic neuritis. Experimental eye research, 87(3); 2008: 208-213.
46. Singer, M., Tan, CS., Bell, D. et al.: Area of peripheral retinal nonperfusion and treatment response in branch and central retinal vein occlusion. Retina, 34; 2014: 1736–42.
47. Stefansson, E., Pedersen, DB., Jensen, PK. et al.: Optic nerve oxygenation. Prog Retin Eye Res, 24; 2005: 307–332.
48. Svrčinová, T., Mareš, J., Chrapek, O. et al.: Changes in oxygen saturation and the retinal nerve fibre layer in patients with optic neuritis – a pilot study. Acta ophthalmol, 96(3); 2018: 309-314.
49. Šínová, I., Chrapek, O., Mlčák, P. et al.: Automatická retinální oxymetrie u pacientů s diabetickou retinopatií. Cesk Slov Oftalmol, 72(5); 2016: 182-186.
50. Šínová, I., Řehák, J., Nekolová, J. et al.: Correlation between ischemic index of retinal vein occlusion and oxygen saturation in retinal vessels. Am J Ophthalmol, 188; 2018: 74-80.
51. Takahashi, K., Muraoka, K., Kishi, S. et al.: Formation of retinochoroidal collaterals in central retinal vein occlusion. Am J Ophthalmol, 126; 1998: 91–99.
52. Tiedeman, JS., Kirk, SE., Srinivas, S. et al.: Retinal oxygen consumption during hyperglycemia in patients with diabetes without retinopathy. Ophthalmology, 105; 1998: 31–36.
53. Tranquart, F., Arsene, S., Aubert-Urena, AS. et al.: Doppler assessment of hemodynamic changes after hemodilution in retinal vein occlusion. J Clin Ultrasound, 26; 1998:119–24.
54. Tranquart, F., Arsene, S., Giraudeau, B. et al.: Initial color Doppler findings in retinal vein occlusion. J Clin Ultrasound, 28; 2000: 28–33.
55. Traustason, S., La Cour, M., Larsen, M.: Retinal vascular oximetry during ranibizumab treatment of central retinal vein occlusion. Br J Ophthalmol, 98; 2014: 1208–1211.
56. Traustason, S., Hardarson, SH., Gottfredsdottir, MS. et al.: Dorzolamide-timolol combination and retinal vessel oxygen saturation in patients with glaucoma or ocular hypertension. Br J Ophthalmol, 93; 2009: 1064–1067.
57. Tsui, I., Kaines, A., Havunjian, MA. et al.: Ischemic index and neovascularization in central retinal vein occlusion. Retina, 231; 2011: 105–110.
58. Williams, R., Airey, M., Baxter, H. et al.: Epidemiology of diabetic retinopathy and macular oedema: a systematic review. Eye (Lond), 18; 2004: 963–983.
59. Williamson, TH., Grewal, J., Gupta, B. et al.: Measurement of PO2 during vitrectomy for central retinal vein occlusion, a pilot study. Graefes Arch Clin Exp Ophthalmol, 247; 2009: 1019–1023.
60. Yoneya, S., Saito, T., Nishiyama, Y. et al.: Retinal oxygen saturation levels in patients with central retinal vein occlusion. Ophthalmology, 109; 2002: 1521–1526.
61. Zong, Y., Lin, L., Yi, C. et al.: Retinal vessel oxygen saturation and vessel diameter in retinitis pigmentosa at various ages.Graefes Arch Clin Exp Ophthalmol, 254(2); 2016: 243-252.
62. Willerslev, A., Hansen, MM., Klefter, O. et al.: Noninvasive imaging of retinal blood flow in myeloproliferative neoplasms. Acta ophthalmol, 95(2); 2017: 146-152.
Štítky
OftalmológiaČlánok vyšiel v časopise
Česká a slovenská oftalmologie
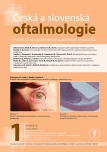
2020 Číslo 1
- Cyklosporin A v léčbě suchého oka − systematický přehled a metaanalýza
- Myasthenia gravis: kombinace chirurgie a farmakoterapie jako nejefektivnější modalita?
- Pomocné látky v roztoku latanoprostu bez konzervačních látek vyvolávají zánětlivou odpověď a cytotoxicitu u imortalizovaných lidských HCE-2 epitelových buněk rohovky
- Konzervační látka polyquaternium-1 zvyšuje cytotoxicitu a zánět spojený s NF-kappaB u epitelových buněk lidské rohovky
- Dlouhodobé výsledky lokální léčby cyklosporinem A u těžkého syndromu suchého oka s 10letou dobou sledování
Najčítanejšie v tomto čísle
- Importance of PET/CT examination in patients with malignant uveal melanoma
- Our experience with micropulse cyclophotocoagulation in the therapy of glaucoma
- Visual Training in Virtual Reality in Adult Patients with Anisometric Amblyopia
- Transscleral Extraction of an Intraocular Foreign Body from the Posterior Segment of the Eye without Pars Plana Vitrectomy