Advantages and limitations of 3D organoids and ex vivo tumor tissue culture in personalized medicine for prostate cancer
Výhody a omezení 3D organoidů a ex vivo kultivace nádorových tkání v personalizované medicíně pro karcinom prostaty
Východiska: Současné in vitro modelové systémy plně neodrážejí biologickou a klinickou diverzitu karcinomu prostaty (prostate cancer – PCa). Organoidy jsou 3D in vitro buněčné kultury, které mohou lépe rekapitulovat heterogenitu onemocnění a zachovat vlastnosti původního nádoru. Krátkodobá ex vivo kultivace PCa tkání může také usnadnit testování léčiv v personalizované medicíně. Materiál a metody: Pro organoidní kultivaci jsme zpracovali jak nádorovou, tak normální tkáň od 50 pacientů, kteří podstoupili radikální prostatektomii nebo transuretrální resekci prostaty. Kromě toho jsme využili techniku ex vivo tkáňové kultivace a provedli krátkodobý experiment s použitím gemcitabinu a inhibitoru Chk1 MU380 ve vzorcích od 10 pacientů. Výsledky: Celkem jsme byli schopni kultivovat organoidy z 58 % nádorových (29/50) a 69 % normálních tkání (20/29). Imunohistochemické barvení dvou reprezentativních případů odhalilo buněčnou pozitivitu na pan-cytokeratin potvrzující přítomnost epiteliálních buněk. Nadměrná exprese proteinů AMACR a ERG v nádorech však nebyla zachována v organoidech. Dalším omezením bylo udržení organoidů pouze do první pasáže, obvykle po dobu 3 týdnů. Dále byly provedeny krátkodobé testy v ex vivo kultuře nádorových tkání od deseti pacientů. Tkáňové vzorky z prostatektomií většinou vykazovaly nízkou míru proliferace a Ki-67 pozitivity. Další nevýhodou tohoto přístupu byla nekonzistentní morfologie mezi jednotlivými tkáňovými fragmenty. Pouze jeden případ vykazoval vysokou míru proliferace pro testování léčiv a nádorová tkáň byla přítomna ve všech testovaných vzorcích. V naší práci také poskytujeme přehled nedávných studií a podrobné srovnání kultivačních podmínek. Závěr: Podařilo se nám ustavit kultury organoidů i fragmentů tkání z primárních nádorů prostaty. Exprese nádorových markerů však nebyla zachována v získaných organoidech. Nekonzistentní morfologie a nízká proliferace ztěžovaly interpretaci výsledků testování léčiv u většiny případů. Přesto mohou být tyto přístupy slibné při použití tkání z metastatického kastračně rezistentního karcinomu prostaty.
Klíčová slova:
karcinom prostaty – personalizovaná medicína – organoidy – tkáňová kultivace ex vivo
Authors:
A. Mickova 1,2; M. Morong 1; M. Levková 1,2; D. Kurfúrstová 1; G. Kharaishvili 1; I. Überall 1; V. Student jr. 3; V. Student 3; S.- Drapela 4 6; K.- Soucek 4 6; J. Bouchal 1,2
Authors place of work:
Department of Clinical and Molecular Pathology, Faculty of Medicine and Dentistry, Palacky University and University Hospital, Olomouc, Czech Republic
1; Institute of Molecular and Translational Medicine, Faculty of Medicine and Dentistry, Palacky University, Olomouc, Czech Republic
2; Department of Urology, University Hospital, Olomouc, Czech Republic
3; Department of Cytokinetics, Institute of Biophysics of the Czech Academy of Sciences, Brno, Czech Republic
4; International Clinical Research Center, St. Anne‘s University Hospital in Brno, Brno, Czech Republic
5; Department of Experimental Biology, Faculty of Science, Masaryk University, Brno, Czech Republic
6
Published in the journal:
Klin Onkol 2022; 35(6): 473-481
Category:
Původní práce
doi:
https://doi.org/10.48095/ccko2022473
Summary
Background: Current in vitro model systems do not fully reflect the biological and clinical diversity of prostate cancer (PCa). Organoids are 3D in vitro cell cultures that may better recapitulate disease heterogeneity and retain parental tumor characteristics. Short-term ex vivo culture of PCa tissues may also facilitate drug testing in personalized medicine. Materials and methods: For organoid culture, we have processed both cancer and normal tissues from 50 patients who underwent radical prostatectomy or transurethral resection of the prostate. In addition, we exploited the ex vivo tissue culture technique and performed short-term chemotherapy assay using gemcitabine and Chk1 inhibitor MU380 in 10 patient samples. Results: In total, we were able to cultivate organoids from 58% of tumors (29/50) and 69% of normal tissue (20/29). Immunohistochemical staining of two representative cases revealed cell positivity for pan-cytokeratin confirming the presence of epithelial cells. However, the overexpression of AMACR and ERG proteins in tumors was not recapitulated in organoids. Another limitation was the propagation of organoids only up to 3 weeks till the first passage. Next, a short-term drug test was performed for ten patients using ex vivo tissue culture. Samples from prostatectomies mostly presented a low proliferation rate as assessed by Ki-67 staining. Another drawback of this approach was inconsistent tissue morphology among particular tissue fragments. Only one case showed a high proliferation rate for drug testing and tumor tissue was present in all tested samples. In our work, we also provide an overview of recent studies and a detailed comparison of culture conditions. Conclusion: We have established cultures of both organoids and tissue fragments from PCa patient samples. However, the expression of tumor markers was not recapitulated in organoids. Inconsistent morphology among tissue fragments and low proliferation hampered the interpretation of the drug testing in most cases. Still, these approaches may be promising using tissues from metastatic castration-resistant prostate cancer.
Keywords:
prostate cancer – Organoids – personalized medicine – ex vivo tissue culture
Introduction
Prostate cancer is the second most frequent cancer as well as the second leading cause of cancer-related deaths in men in Western countries [1]. This malignancy develops as androgen-dependent and initially responds to androgen deprivation therapies; however, it ultimately progresses to incurable castration-resistant stage accompanied with metastatic dissemination to the bones, lung, brain, or liver. Personalized medicine may help in the next therapy selection for these patients with progressive disease. The application of next generation sequencing and armamentarium of new treatment options for castration resistant prostate cancer (CRPC) patients have recently been reviewed [2,3]. Importantly, therapy decision making may also be facilitated by ex vivo drug testing.
Suitable models for this purpose may be self-organized three-dimensional organoid cell cultures that should largely recapitulate heterogeneous composition of original tumor and preserve gene mutation landscape associated with the cancer development [4–7]. Clevers et al were the first who established cell culture of genetically stable prostate organoids derived from luminal and basal adult stem cells [8,9]. Importantly, they introduced necessary factors promoting mitosis, Wnt pathway activation, and bone morphogenetic protein (BMP) pathway inhibition, that ensured the growth of stem cells [4]. The ability to grow organoids with high efficiency from healthy adult human stem cells paved the way for the establishment of organoids from patient-derived tumor tissue [10]. So far, it has been shown that organoid cultures keep mutational patterns during long-term cultivation without genetic alterations in several cancer types such as colon, esophagus, pancreas, stomach, liver, endometrium, and breast [4,11–20]. Similar results were achieved using metastatic colon and breast bioptic samples [6,10,20]. This cell culture approach has also been tested for PCa in several studies [21–24]. Another approach for personalized drug screening is represented by short-term ex vivo tissue culture. This model retains original tissue architecture but enables only short-term drug testing. It has been previously utilized for multiple tumor types including prostate, bladder, breast, colon, or gastric cancer [25–30].
Taken together, both organoids and ex vivo tissue cultures can serve as clinically relevant tools for improved therapy decision making for patients with advanced prostate cancer (Fig. 1). This study aimed to establish organoid and ex vivo tissue culture models and determine their advantages and limitations.
Methods
Patient selection and tissue collection
The study was approved by the Ethical Committee of the University Hospital Olomouc (Ref. No. 127/14) and all experimental procedures were done in compliance with the Declaration of Helsinki, as well as with the law of the Czech Republic. All human samples were obtained at the Department of Urology of the University Hospital (Olomouc, Czech Republic), based on informed consent signed by all patients involved in the study. The patient’s cohort consists of patients who have undergone radical prostatectomy or TURP (transurethral resection of the prostate) in the years 2017–2020. Tumor and benign samples were chosen by the skilled pathologists (G.K., D.K., and M.M.).
Establishment and cultivation of organoids or spheroids
Tissue specimens were placed into a sterile Petri dish, washed three times with 1× phosphate buffer solution (PBS), and minced into small pieces. Three approaches were used for tissue digestion. First, the tissue was dissociated by Collagenase I-A (2.5 mg/mL, Sigma-Aldrich, St. Louis, USA) for 2 hours. Second, the tissue was dissociated by Collagenase Type I (125 units/ml, Worthington Biochemical Corp., Lakewood, USA) overnight and additionally retrieved by Tryple (Sigma-Aldrich, St. Louis, USA) for 15 min. Third, the subset of tissue samples was dissociated by Tumor Cell Isolation Kit and gentleMACS Dissociator (Miltenyi Biotec, Germany) according to the manufacturer’s protocol.
The single-cell suspension (50 thousand) was seeded into the drop of Matrigel (Corning; USA) in a Nunc 4-well dish IVF (Thermo Scientific, USA) or in a 24-well low-attachment plate (Corning; USA). The main subset of samples was cultured according to [31]. Advanced DMEM/ F-12 medium (ThermoFisher, Waltham, USA) was supplemented with 1× Glutamax (ThermoFisher, Waltham, USA), 10 mM HEPES (ThermoFisher, Waltham, USA), 1% antibiotics and enriched with 1× B27 supplement (ThermoFisher, Waltham, USA), 5 ng/ml EGF (ThermoFisher, Waltham, USA), 5 ng/mL FGF2 (Peprotech, London, UK), 10 ng/mL FGF10 (Peprotech, London, UK), 10 mM nicotinamide (Sigma-Aldrich, St. Louis, USA), 500 nM A83-01 (Tocris Bioscience, Bristol, UK), 10 µM SB 202 190 (Sigma-Aldrich, St. Louis, USA), 1 µM prostaglandin E2 (Tocris Bioscience, Bristol, UK), 1 nM R1881 (Organon, Wien, Austria), 1.25 mM N-acetylcysteine (Sigma-Aldrich, St. Louis, USA), 10 µM ROCK- inhibitor Y-27632 dihydrochloride (Tab. 1). Importantly, the medium was also supplemented either with 0.5 µg/mL recombinant R-spondin 1 and 0.1 µg/mL noggin (both Peprotech, London, UK) or with respective conditioned media (see below and Suppl. Tab. 1). A subset of samples was simultaneously cultured in a modified medium without nicotinamide and inhibitor of p38 (SB 202 190) (Suppl. Tab. 1) [32]. We have also adopted spheroid and organoid culture [22]. For a total volume of 100 mL, this StemPro medium was composed of 89.8 mL Gibco GlutaMAX DMEM/F-12 (ThermoFisher, Waltham, USA), 7.2 mL 25% BSA (ThermoFisher, Waltham, USA), 182 µL 2-Mercaptoethanol (ThermoFisher, Waltham, USA), 2 mL StemPro hESC Supplement (ThermoFisher, Waltham, USA), 1 mL penicillin/streptomycin (Sigma-Aldrich, St. Louis, USA), 1 µL 10 µM R1881 (Organon, Wien, Austria), 40 µL FGF-b (20 µg/mL; ThermoFisher, Waltham, USA), and 17.48 µL 50 mM Y-27632 (Santa Cruz Biotechnology, USA). The organoids in Matrigel were passaged via trituration and dissociation using Dispase (Thermo Fisher, Waltham, MA, USA). Cell suspensions from the organoids or spheroids were further dissociated by Liberase (Roche, Basel, Switzerland) or Tryple (Sigma-Aldrich, St. Louis, MO, USA) [33].
R-spondin 1 and noggin conditioned medium
The HEK 293-T cells producing R-spondin 1 and noggin were cultured as previously described [21]. The cells produced R-spondin 1 and noggin for 7 days in serum-free ADMEM (Advanced Dulbecco‘s modified eagle medium; Sigma-Aldrich, St. Louis, MO, USA) supplemented with HEPES and Glutamax. The activity of the conditioned R-spondin 1 was confirmed by the increase of luciferase signal using SuperTopFlash (STF) 293T cells. Twenty percent of conditioned R-spondin 1 media in the complete medium was equal to 0.5 µg/mL recombinant R-spondin 1 (Peprotech) (Suppl. Fig. 1). The activity of the noggin conditioned medium was confirmed by the decrease of luciferase signal after the addition of 0.1 µg/mL BMP4 to BMP reporter cell line, BRITER (BMP Responsive Immortalized Reporter cell line; purchased from Kerafast, USA) [34]. Twenty percent of conditioned noggin in the complete medium corresponded to the activity of 0.1 µg/mL recombinant noggin (Peprotech) (Suppl. Fig. 2).
Paraffin-embedding of organoids and spheroids and immunohistochemistry
The pellet of spheroids was resuspended in the mixture of 225 µL plasma (blood was collected in sodium citrate vacuum tubes from volunteers, spun 5 min at 3 000 g, and the supernatant was frozen in aliquots) with 5.65 µL of 1 M calcium chloride. A clot was formed by adding 22.5 µL thrombin (120 NIHU/mL, Sigma Aldrich, St. Louis, MO, USA) and transferred with a pipette tip into Tissue-Tek Paraform Sectionable Cassette for small samples (Sakura, The Netherlands). The organoids in Matrigel were directly placed into the same cassette using an iron spoon. Formalin-fixation and paraffin-embedding (FFPE) were performed according to standard protocol. FFPE organoids, spheroids, and tissue samples were immunostained with appropriate antibodies according to the standard manual or automatic protocols (Suppl. Tab. 2).
Human prostate cancer tissue culture
We have adopted the Knudsen protocol for short-term ex vivo tissue culture [25,27]. Briefly, fresh tissue was obtained from a pathologist immediately following radical prostatectomy. Under a laminar flow hood, the tissue was placed into the lid of a 10-cm plate and dissected into 1-mm3 pieces with a scalpel. The pieces of tissue were placed in 24-well plates on sponges (Spongostan dental, Johnson & Johnson), which were soaked in 500 µL media (DMEM/F12 supplemented with 5% heat-inactivated fetal bovine serum (FBS), hydrocortisone, insulin from bovine pancreas, and 100 units/mL penicillin-streptomycin) and appropriate treatment at time 0 (vehicle or 500 nM gemcitabine, purchased from Carbosynth Ltd, Compton, UK). The plates were placed in an incubator at 37 °C and 5% CO2. The treatment with 4 μM MU380 was performed 24 hours later, and the tissues were harvested at a time point of 48 hours [35,36]. The experimental design was the same as in our previous in vitro and animal experiments [35]. From each treatment, two pieces were frozen and one was fixed in 4% formalin. The formalin-fixed and paraffin-embedded blocks were cut with a microtome and the slides underwent standard hematoxylin and eosin staining. The samples with cancer cells present in all experimental conditions were immunohistochemically stained for Ki67 and γH2AX.
Results
Establishment of 3D organoids from tumor and normal tissues
We have processed normal and cancer tissue from 50 patients who underwent either radical prostatectomy or transurethral resection of the prostate (TURP). Their median age was 65 (range 46–85) years and the median Gleason score was 7 (range 7–10) (Suppl. Tab. 1). Several prostate organoid culture conditions have so far been used [21–23,32,37]. The overview of various growth factors is summarized in Tab. 1. We have adopted the protocol by Drost et al, with higher efficiency of organoid growth in the presence of recombinant noggin (77% of both tumor and non-tumor samples) in comparison to the noggin-conditioned media (33% and 41% of the tumor and non-tumor samples, respectively; Suppl. Tab. 1) [21,31]. Two representative cases are shown in Fig. 2 and Suppl. Fig. 3. The activity of conditioned media for both noggin and R-spondin 1 was determined by luciferase assays (Suppl. Fig. 1 and 2). To achieve better organoid growth, we modified this medium, with exclusion of nicotinamide and inhibitor of p38 (SB 202 190) [32]. We observed significant increase in growth in the modified medium which was documented by a larger radius of organoids (Suppl. Fig. 4A). On the other hand, none of the above-mentioned modifications ensured the growth of tumor cells (Suppl. Fig. 4B) and long-term organoid culture. We have also adopted a protocol by Linxweiler et al for spheroid and organoid cultures [22]. The success rate was comparable with previous culture conditions being 50% (4/8) and 77% (7/9) for spheroid and organoid cultures, respectively. In total, we were able to cultivate organoids from 58% of tumors (29/50) and from 69% of normal tissue (20/29). We did not observe any association between Gleason score and organoid formation. The samples with no significant increase in size 7 days after seeding were considered as non-proliferative. The average length of cultivation was 21 days; however, we were not successful in subsequent passaging and long-term cultivation.
Immunohistochemical analysis of prostate organoids
Two representative cases (no. 1595 and 1558) were selected for the comparative analysis of selected proteins in organoids and primary patient tissue (Fig. 2 and Suppl. Fig. 3). Their pathological stage was pT3a and pT2b with the Gleason score 9 and 7, respectively, both without metastasis in the lymph nodes. Whole organoid immunohistochemical staining of two representative cases revealed positivity for pan-cytokeratin confirming the presence of epithelial cells. Both cases were partially positive for the androgen receptor and p63 protein. The fraction of cells from the first case (Fig. 2) was positive for luminal marker, cytokeratin 18, while the other case (Suppl. Fig. 3) was positive for cytokeratin 19, a marker of intermediate cells. However, overexpression of AMACR and ERG proteins was not recapitulated in tumor organoids.
Short-term drug testing in ex vivo tissue culture.
Despite intensive efforts in different laboratories, the efficiency of PCa organoid culture is low [21,22,24]. An alternative approach for personalized medicine is short-term tissue fragment culture. We have adopted the Knudsen protocol for small tissue pieces on a sponge for the testing of a Chk1 inhibitor in combination with gemcitabine [25,27,35,36]. Ten prostatectomy samples were processed (Suppl. Tab. 3); however, most cases displayed either low proliferation rate or missing cancer cells in selected tissue pieces. Only one experiment provided reasonable results with cancer cells in all tissues tested. The combined treatment of Chk1 inhibitor and gemcitabine inhibited proliferation and induced substantial DNA damage, as assessed by Ki67 and γH2AX staining, respectively (Fig. 3). The observed advantages and limitations of organoid and tissue culture are summarized in Tab. 2.
Discussion
Our goal was to establish conditions for the cultivation of primary PCa samples that can pave the way for the analysis of biopsies from castration-resistant patients, allowing them to potentially benefit from drug screening. Cancer cell lines derived from primary patient material have contributed tremendously to cancer research, however, they have several drawbacks. For example, their generation from primary patient material is inefficient and involves selection to in vitro 2D culture conditions. Furthermore, only rare clones are able to expand and no longer recapitulate the heterogeneity of the original tumors [10]. Patient-derived xenografts (PDX) are considered as a gold-standard for cancer research and preclinical drug testing; however, this approach is expensive and time consuming, and PDXs may undergo mouse-specific tumor evolution [10,38,39]. Organoids and ex vivo tissue culture have been proposed as suitable alternative models.
Since the culture conditions for prostate tumor organoids are not strictly defined, we have summarized the work published so far in Tab. 1. By adopting the protocol by Drost et al, we were able to cultivate 77% of both tumor and non-tumor samples in our laboratory conditions [31]. The organoids grew more efficiently in the presence of recombinant noggin in comparison to the conditioned medium. These culture conditions supported the growth of epithelial cells; nevertheless, we were unable to confirm the growth of tumor cells with AMACR or ERG overexpression in two representative cases. Other researchers have also been unable to establish primary tumor organoids, as less aggressive specimens are prone to overgrowth from normal prostate epithelium in biopsy samples [21,40]. Another disadvantage was the length of cultivation, which did not last longer than 3 weeks, until the first passage. Therefore, we have tested further modifications of the protocol. Recent work suggested leaving out p38 inhibitor SB202190 and nicotinamide from the medium [32]. We had indeed observed larger organoids using this modification; however, neither AMACR- nor ERG-positive tumor cells were detected. Linxweiler et al reported spheroid culture from organ-confined PCa, including short-term drug testing [22]. However, we have not observed improved growth of neither spheroids nor organoids in the reported medium. One of the reasons may be limited material amount used as an input and the presence of non-tumor cells.
Comprehensive analysis of organoids from 81 clinical specimens cultured in the presence of R-spondin 1 and noggin has been published recently [24]. Despite initial success in the tumor organoid establishment, all were progressively overgrown by normal cells and gained a benign-like phenotype. Authors performed IHC analysis of ERG and PTEN at early time points (up to 30 days). Notably, none of the four organoid cultures derived from samples with ERG overexpression and PTEN positivity exhibited positivity for ERG. The authors also processed nine metastatic samples and established one novel organoid model derived from hormone-naïve lung metastasis. A recent study pointed out the essential role of stromal paracrine signaling on organoid growth and phenotype [23]. Co-cultivation with patient-derived stromal cells caused increased branching and budding of prostate epithelial organoids, that greatly mimicked the normal in vivo acinar structure. Importantly, the organoids derived from areas of PCa maintained expression of AMACR and showed increased viability. Stromal cells expressed a plethora of factors, which were not observed in non-prostatic feeder fibroblasts.
Another approach for short-term drug screening is the whole tissue culture, which maintains all components of the tumor microenvironment. The tumor cells in this model system exhibited de novo proliferative capacity that can be used to predict therapeutic response and identify novel targets in advanced disease [27]. We have adopted a protocol for small tissue pieces on a sponge [25,27] for testing of a Chk1 inhibitor in combination with gemcitabine. We showed that treatment of tumor tissue with the combination of both drugs results in decreased proliferation and induction of DNA damage, as assessed by Ki67 and γH2AX staining, respectively. These results are in line with in vitro and in vivo experiments in our previous study [36]. On the other hand, most of the cases displayed either low proliferation rate or missing cancer cells in selected tissue pieces. This could be improved by the preparation of consecutive sections using vibratome, a device invented for cutting fresh tissues. Low--rate proliferation is typical for primary prostate tumors; however, it is expected high in samples from CRPC patients, in particular from metastases. Despite the multiclonality and heterogeneity of primary cancer, clonal bottlenecks imposed by the metastatic process and further by therapeutic interventions seem to select for a single dominant clone in lethal metastatic PCa [41]. Several studies have recently used tissue slices prepared by vibratome for drug testing, including enzalutamide, apalutamide, docetaxel, gemcitabine, or olaparib [28,42,43]. This methodology has also been used for the analysis of tumor aggressiveness under hypoxia conditions [44]. Tissue slicing and ex vivo culture may also be used for PDX which are considered as a gold-standard for cancer research and preclinical drug testing [38,39]. The faithful retention of tissue structure and function in ex vivo PDX culture offers an ideal opportunity for treatment efficacy screening, thereby reducing costs and numbers of experimental animals [39,42].
Conclusion
We have established a culture of both organoids and tissue fragments from patients with primary PCa. However, the organoids did not fully recapitulate primary tissue characteristics and heterogeneity between tissue fragments hampered interpretation of the drug testing. Still, these approaches may be promising for the establishment of organoids and ex vivo tissue fragment cultures using tissues from metastatic castration-resistant PCa.
Acknowledgements
The HEK 293-T cells producing R-spondin 1 and noggin were kindly provided by prof. Calvin Kuo (Stanford University, USA) and Hans Clevers (Hubrecht Institute, Netherlands), respectively. SuperTopFlash (STF) 293T cells were kindly provided by Dr. Vitezslav Bryja (Masaryk University, Czechia). Chk1 inhibitor MU380 was kindly provided by Dr. Kamil Paruch (Masaryk University, Czechia)
Funding
This work was supported in part by the Czech Ministry of Health (grants NU20-03-00201 and DRO (FNOL, 00098892)), Palacky University (LF_2021_005), the European Regional Development Fund (ERDF) and MEYS CR (projects CZ.02.1.01/0.0/0.0/16_019/0000868 – Enoch, LM2018130 – CZ-OPENSCREEN: National Infrastructure for Chemical Biology, and CZ.02.1.01/0.0/0.0/16_013/0001674 – BBMRI-CZ).
MUDr. Daniela Kurfürstová, Ph.D.
Ústav klinické a molekulární patologie
LF UP a FN Olomouc
Hněvotínská 3
775 15 Olomouc
e-mail: daniela.kurfurstova@fnol.cz
doc. Mgr. Jan Bouchal, Ph.D.
Ústav klinické a molekulární patologie
LF UP a FN Olomouc
Hněvotínská 3
775 15 Olomouc
e-mail: jan.bouchal@upol.cz
Submitted/Obdrženo: 19. 10. 2021
Accepted/Přijato: 13. 6. 2022
For supplementary figures and tables, see the online version of the article at www.linkos.cz./ casopis-klinicka-onkologie/
Zdroje
1. Siegel RL, Miller KD, Fuchs HE et al. Cancer Statistics, 2021. CA Cancer J Clin 2021; 71 (1): 7–33. doi: 10.3322/ caac.21654.
2. Lakshmanan V-K, Ojha S, Jung YD. A modern era of personalized medicine in the diagnosis, prognosis, and treatment of prostate cancer. Comput Biol Med 2020; 126: 104020. doi: 10.1016/j.compbiomed.2020.104 020.
3. Norz V, Rausch S. Treatment and resistance mechanisms in castration-resistant prostate cancer: new implications for clinical decision making? Expert Rev Anticancer Ther 2021; 21 (2): 149–163. doi: 10.1080/14737140. 2021.1843430.
4. Xia X, Li F, He J et al. Organoid technology in cancer precision medicine. Cancer Lett 2019; 457: 20–27. doi: 10.1016/j.canlet.2019.04.039.
5. Pauli C, Hopkins BD, Prandi D et al. Personalized in vitro and in vivo cancer models to guide precision medicine. Cancer Discov 2017; 7 (5): 462–477. doi: 10.1158/2159-8290.CD-16-1154.
6. Weeber F, van de Wetering M, Hoogstraat M et al. Preserved genetic diversity in organoids cultured from biopsies of human colorectal cancer metastases. Proc Natl Acad Sci U S A 2015; 112 (43): 13308–13311. doi: 10.1073/pnas.1516689112.
7. Kretzschmar K, Clevers H. Organoids: modeling development and the stem cell niche in a dish. Dev Cell 2016; 38 (6): 590–600. doi: 10.1016/j.devcel.2016.08.014.
8. Karthaus WR, Iaquinta PJ, Drost J et al. Identification of multipotent luminal progenitor cells in human prostate organoid cultures. Cell 2014; 159 (1): 163–175. doi: 10.1016/j.cell.2014.08.017.
9. Sato T, Vries RG, Snippert HJ et al. Single Lgr5 stem cells build crypt-villus structures in vitro without a mesenchymal niche. Nature 2009; 459 (7244): 262–265. doi: 10.1038/nature07935.
10. Drost J, Clevers H. Organoids in cancer research. Nat Rev Cancer 2018; 18 (7): 407–418. doi: 10.1038/s41568-018-0007-6.
11. Schütte M, Risch T, Abdavi-Azar N et al. Molecular dissection of colorectal cancer in pre-clinical models identifies biomarkers predicting sensitivity to EGFR inhibitors. Nat Commun 2017; 8: 14262. doi: 10.1038/ncomms 14262.
12. Sato T, Stange DE, Ferrante M et al. Long-term expansion of epithelial organoids from human colon, adenoma, adenocarcinoma, and Barrett’s epithelium. Gastroenterology 2011; 141 (5): 1762–1772. doi: 10.1053/j.gastro.2011.07.050.
13. Van de Wetering M, Francies HE, Francis JM et al. Prospective derivation of a living organoid biobank of colorectal cancer patients. Cell 2015; 161 (4): 933–945. doi: 10.1016/j.cell.2015.03.053.
14. Fujii M, Shimokawa M, Date S et al. A colorectal tumor organoid library demonstrates progressive loss of niche factor requirements during tumorigenesis. Cell Stem Cell 2016; 18 (6): 827–838. doi: 10.1016/j.stem.2016.04.003.
15. Boj SF, Hwang C-I, Baker LA et al. Organoid models of human and mouse ductal pancreatic cancer. Cell 2015; 160 (1–2): 324–338. doi: 10.1016/j.cell.2014.12.021.
16. Huang L, Holtzinger A, Jagan I et al. Ductal pancreatic cancer modeling and drug screening using human pluripotent stem cell- and patient-derived tumor organoids. Nat Med 2015; 21 (11): 1364–1371. doi: 10.1038/nm.3973.
17. Bartfeld S, Bayram T, van de Wetering M et al. In vitro expansion of human gastric epithelial stem cells and their responses to bacterial infection. Gastroenterology 2015; 148 (1): 126–136.e6. doi: 10.1053/j.gastro.2014.09. 042.
18. Broutier L, Mastrogiovanni G, Verstegen MM et al. Human primary liver cancer-derived organoid cultures for disease modeling and drug screening. Nat Med 2017; 23 (12): 1424–1435. doi: 10.1038/nm.4438.
19. Turco MY, Gardner L, Hughes J et al. Long-term, hormone-responsive organoid cultures of human endometrium in a chemically defined medium. Nat Cell Biol 2017; 19 (5): 568–577. doi: 10.1038/ncb3516.
20. Sachs N, de Ligt J, Kopper O et al. A living biobank of breast cancer organoids captures disease heterogeneity. Cell 2018; 172 (1–2): 373–386.e10. doi: 10.1016/j.cell.2017.11.010.
21. Gao D, Vela I, Sboner A et al. Organoid cultures derived from patients with advanced prostate cancer. Cell 2014; 159 (1): 176–187. doi: 10.1016/j.cell.2014.08.016.
22. Linxweiler J, Hammer M, Muhs S et al. Patient-derived, three-dimensional spheroid cultures provide a versatile translational model for the study of organ-confined prostate cancer. J Cancer Res Clin Oncol 2019; 145 (3): 551–559. doi: 10.1007/s00432-018-2803-5.
23. Richards Z, McCray T, Marsili J et al. Prostate stroma increases the viability and maintains the branching phenotype of human prostate organoids. iScience 2019; 12: 304–317. doi: 10.1016/j.isci.2019.01.028.
24. Servant R, Garioni M, Vlajnic T et al. Prostate cancer patient-derived organoids: detailed outcome from a prospective cohort of 81 clinical specimens. J Pathol 2021; 254 (5): 543–555. doi: 10.1002/path.5698.
25. Schiewer MJ, Goodwin JF, Han S et al. Dual roles of PARP-1 promote cancer growth and progression. Cancer Discov 2012; 2 (12): 1134–1149. doi: 10.1158/2159-8290.CD-12-0120.
26. Naipal KAT, Verkaik NS, Sánchez H et al. Tumor slice culture system to assess drug response of primary breast cancer. BMC Cancer 2016; 16: 78. doi: 10.1186/s12885-016-2119-2.
27. Shafi AA, Schiewer MJ, de Leeuw R et al. Patient-derived models reveal impact of the tumor microenvironment on therapeutic response. Eur Urol Oncol 2018; 1 (4): 325–337. doi: 10.1016/j.euo.2018.04.019.
28. Van de Merbel AF, van der Horst G, van der Mark MH et al. An ex vivo tissue culture model for the assessment of individualized drug responses in prostate and bladder cancer. Front Oncol 2018; 8: 400. doi: 10.3389/fonc.2018.00400.
29. Martin SZ, Wagner DC, Hörner N et al. Ex vivo tissue slice culture system to measure drug-response rates of hepatic metastatic colorectal cancer. BMC Cancer 2019; 19 (1): 1030. doi: 10.1186/s12885-019-6270-4.
30. Chen S, Chen C, Hu Y et al. Three-dimensional ex vivo culture for drug responses of patient-derived gastric cancer tissue. Front Oncol 2020; 10: 614096. doi: 10.3389/fonc.2020.614096.
31. Drost J, Karthaus WR, Gao D et al. Organoid culture systems for prostate epithelial and cancer tissue. Nat Protoc 2016; 11 (2): 347–358. doi: 10.1038/nprot.2016. 006.
32. Beshiri ML, Tice CM, Tran C et al. A PDX/organoid biobank of advanced prostate cancers captures genomic and phenotypic heterogeneity for disease modeling and therapeutic screening. Clin Cancer Res 2018; 24 (17): 4332–4345. doi: 10.1158/1078-0432.CCR-18-0409.
33. Strnadel J, Woo SM, Choi S et al. 3D culture protocol for testing gene knockdown efficiency and cell line derivation. Bio Protoc 2018; 8 (11): e2874. doi: 10.21769/BioProtoc.2874.
34. Yadav PS, Prashar P, Bandyopadhyay A. BRITER: a BMP responsive osteoblast reporter cell line. PLoS One 2012; 7 (5): e37134. doi: 10.1371/journal.pone.0037134.
35. Samadder P, Aithal R, Belan O et al. Cancer TARGETases: DSB repair as a pharmacological target. Pharmacol Ther 2016; 161: 111–131. doi: 10.1016/j.pharmthera.2016.02.007.
36. Drápela S, Khirsariya P, van Weerden WM et al. The CHK1 inhibitor MU380 significantly increases the sensitivity of human docetaxel-resistant prostate cancer cells to gemcitabine through the induction of mitotic catastrophe. Mol Oncol 2020; 14 (10): 2487–2503. doi: 10.1002/1878-0261.12756.
37. Liu X, Ory V, Chapman S et al. ROCK inhibitor and feeder cells induce the conditional reprogramming of epithelial cells. Am J Pathol 2012; 180 (2): 599–607. doi: 10.1016/j.ajpath.2011.10.036.
38. Navone NM, van Weerden WM, Vessella RL et al. Movember GAP1 PDX project: an international collection of serially transplantable prostate cancer patient-derived xenograft (PDX) models. Prostate 2018; 78 (16): 1262–1282. doi: 10.1002/pros.23701.
39. Van de Merbel AF, van der Horst G, van der Pluijm G. Patient-derived tumour models for personalized therapeutics in urological cancers. Nat Rev Urol 2021; 18 (1): 33–45. doi: 10.1038/s41585-020-00389-2.
40. Gleave AM, Ci X, Lin D et al. A synopsis of prostate organoid methodologies, applications, and limitations. Prostate 2020; 80 (6): 518–526. doi: 10.1002/pros.23966.
41. Haffner MC, Zwart W, Roudier MP et al. Genomic and phenotypic heterogeneity in prostate cancer. Nat Rev Urol 2021; 18 (2): 79–92. doi: 10.1038/s41585-020-00400-w.
42. Zhang W, Liao C-Y, Chtatou H et al. Apalutamide sensitizes prostate cancer to ionizing radiation via inhibition of non-homologous end-joining DNA repair. Cancers (Basel) 2019; 11 (10): 1593. doi: 10.3390/cancers11101593.
43. Zhang W, van Weerden WM, de Ridder CMA et al. Ex vivo treatment of prostate tumor tissue recapitulates in vivo therapy response. Prostate 2019; 79 (4): 390–402. doi: 10.1002/pros.23745.
44. Bery F, Figiel S, Kouba S et al. Hypoxia promotes prostate cancer aggressiveness by upregulating EMT-activator Zeb1 and SK3 channel expression. Int J Mol Sci 2020; 21 (13): 4786. doi: 10.3390/ijms21134786.
45. Bery F, Figiel S, Kouba S et al. Hypoxia promotes prostate cancer aggressiveness by upregulating EMT-activator Zeb1 and SK3 channel expression. Int J Mol Sci 2020; 21 (13): 4786. doi: 10.3390/ijms21134786.
Štítky
Detská onkológia Chirurgia všeobecná OnkológiaČlánok vyšiel v časopise
Klinická onkologie
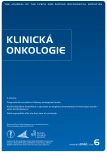
2022 Číslo 6
- Metamizol jako analgetikum první volby: kdy, pro koho, jak a proč?
- Nejasný stín na plicích – kazuistika
- Kombinace metamizol/paracetamol v léčbě pooperační bolesti u zákroků v rámci jednodenní chirurgie
- Fixní kombinace paracetamol/kodein nabízí synergické analgetické účinky
- Antidepresivní efekt kombinovaného analgetika tramadolu s paracetamolem
Najčítanejšie v tomto čísle
- Prognostické a prediktivní faktory meningeomů mozku
- Advantages and limitations of 3D organoids and ex vivo tumor tissue culture in personalized medicine for prostate cancer
- Fatal myocarditis after the first dose of nivolumab
- Fekální mikrobiální transplantace – nová možnost ovlivnění výsledků terapie onkologických pacientů