Endoplasmic Reticulum Chaperones at the Tumor Cell Surface and in the Extracellular Space
Authors:
V. Brychtová; B. Vojtěšek
Authors place of work:
Regionální centrum aplikované molekulární onkologie, Masarykův onkologický ústav, Brno
Published in the journal:
Klin Onkol 2016; 29(Supplementum 4): 25-30
Category:
Review
doi:
https://doi.org/10.14735/amko20164S25
Summary
Background:
Endoplasmic reticulum chaperones are stress induced proteins capable of translocation into cytosol, cell membrane or extracellular space. The chaperones are transported from the endoplasmic reticulum particularly under endoplasmic reticulum stress conditions, while their constitutive extracellular expression was found in many cancers. Cell surface or extracellular endoplasmic reticulum chaperones take up distinct functions compared to their endoplasmic reticulum resident variants because they act like multifunctional receptors and thus affect cell signaling and proliferation.
Aim:
The presented review focuses primarily on endoplasmic reticulum chaperones expression on the cell surface of cancer cells and into extracellular space. The work describes possible mechanisms of chaperones translocation to the cancer cell surface, including KDEL transport mechanism and retrotranslocation and the influence of chaperone posttranslation modifications on their localization within the cell. Well described cancer cell surface endoplasmic reticulum chaperones include GRP78, GRP94, calreticulin and calnexin that are involved in cancer cell signaling in different ways. The attention is also paid to immunogenic properties of membrane-localized chaperones for their ability to participate in immune reactions. They can take part in innate and adaptive immune response through their interaction with toll-like receptors or during the antigen presentation as well as in tumor-specific immunity. The expression of endoplasmic reticulum chaperones on the cancer cells surface is potentially exploitable in specific antitumor therapy as well as vaccine therapy, thus the final part of this review is dedicated to this topic.
Key words:
endoplasmic reticulum – glucose-regulated proteins – molecular chaperones – KDEL sequence – immunobiology
This work was supported by the project MEYS – NPS I – LO1413.
The authors declare they have no potential conflicts of interest concerning drugs, products, or services used in the study.
The Editorial Board declares that the manuscript met the ICMJE recommendation for biomedical papers.
Submitted:
17. 5. 2016
Accepted:
20. 7. 2016
Zdroje
1. Gidalevitz T, Stevens F, Argon Y. Orchestration of secretory protein folding by ER chaperones. Biochim Biophys Acta 2013; 1833 (11): 2410–2424. doi: 10.1016/j.bbamcr.2013.03.007.
2. Lee AS. Glucose-regulated proteins in cancer: molecular mechanisms and therapeutic potential. Nat Rev Cancer 2014; 14 (4): 263–276. doi: 10.1038/nrc3701.
3. Shin BK, Wang H, Yim AM et al. Global profiling of the cell surface proteome of cancer cells uncovers an abundance of proteins with chaperone function. J Biol Chem 2003; 278 (9): 7607–7616.
4. Arap MA, Lahdenranta J, Mintz PJ et al. Cell surface expression of the stress response chaperone GRP78 enables tumor targeting by circulating ligands. Cancer Cell 2004; 6 (3): 275–284.
5. Kim Y, Lillo AM, Steiniger SC et al. Targeting heat shock proteins on cancer cells: selection, characterization, and cell-penetrating properties of a peptidic GRP78 ligand. Biochemistry 2006; 45 (31): 9434–9444.
6. Liu Y, Steiniger SC, Kim Y et al. Mechanistic studies of a peptidic GRP78 ligand for cancer cell-specific drug delivery. Mol Pharm 2007; 4 (3): 435–447.
7. Gonzalez-Gronow M, Selim MA, Papalas J et al. GRP78: a multifunctional receptor on the cell surface. Antioxid Redox Signal 2009; 11 (9): 2299–2306. doi: 10.1089/ARS.2009.2568.
8. Gray PC, Vale W. Cripto/GRP78 modulation of the TGF-beta pathway in development and oncogenesis. FEBS Lett 2012; 586 (14): 1836–1845. doi: 10.1016/j.febslet.2012.01.051.
9. Ni M, Zhang Y, Lee AS. Beyond the endoplasmic reticulum: atypical GRP78 in cell viability, signalling and therapeutic targeting. Biochem J 2011; 434 (2): 181–188. doi: 10.1042/BJ20101569.
10. Sato M, Yao VJ, Arap W et al. GRP78 signaling hub a receptor for targeted tumor therapy. Adv Genet 2010; 69: 97–114. doi: 10.1016/S0065-2660 (10) 69006-2.
11. Horvath I, Multhoff G, Sonnleitner A et al. Membrane-associated stress proteins: more than simply chaperones. Biochim Biophys Acta 2008; 1778 (7–8): 1653–1664. doi: 10.1016/j.bbamem.2008.02.012.
12. Munro S, Pelham HR. A C-terminal signal prevents secretion of luminal ER proteins. Cell 1987; 48 (5): 899–907.
13. Raykhel I, Alanen H, Salo K et al. A molecular specificity code for the three mammalian KDEL receptors. J Cell Biol 2007; 179 (6): 1193–1204.
14. Capitani M, Sallese M. The KDEL receptor: new functions for an old protein. FEBS Lett 2009; 583 (23): 3863–3871. doi: 10.1016/j.febslet.2009.10.053.
15. Basu S, Binder RJ, Ramalingam T et al. CD91 is a common receptor for heat shock proteins gp96, hsp90, hsp70, and calreticulin. Immunity 2001; 14 (3): 303–313.
16. Arispe N, De Maio A. ATP and ADP modulate a cation channel formed by Hsc70 in acidic phospholipid membranes. J Biol Chem 2000; 275 (40): 30839–30843.
17. Voos WK, Rottgers K. Molecular chaperones as essential mediators of mitochondrial biogenesis. Biochim Biophys Acta 2002; 1592 (1): 51–62.
18. Philpott A, Krude T, Laskey RA. Nuclear chaperones. Semin Cell Dev Biol 2000; 11 (1): 7–14.
19. Wiersma VR, Michalak M, Abdullah TM et al. Mechanisms of translocation of ER chaperones to the cell surface and immunomodulatory roles in cancer and autoimmunity. Front Oncol 2015; 5: 7. doi: 10.3389/fonc.2015.00007.
20. Cancino J, Jung JE, Luini A. Regulation of Golgi signaling and trafficking by the KDEL receptor. Histochem Cell Biol 2013; 140 (4): 395–405. doi: 10.1007/s00418-013-1130-9.
21. Marzec M, Eletto D, Argon Y. GRP94: an HSP90-like protein specialized for protein folding and quality control in the endoplasmic reticulum. Biochim Biophys Acta 2012; 1823 (3): 774–787. doi: 10.1016/j.bbamcr.2011.10.013.
22. De Maio A, Vazquez D. Extracellular heat shock proteins: a new location, a new function. Shock 2013; 40 (4): 239–246. doi: 10.1097/SHK.0b013e3182a185ab.
23. Stolz A, Wolf DH. Endoplasmic reticulum associated protein degradation: a chaperone assisted journey to hell. Biochim Biophys Acta 2010; 1803 (6): 694–705. doi: 10.1016/j.bbamcr.2010.02.005.
24. Jakobsen CG, Rasmussen N, Laenkholm AV et al. Phage display derived human monoclonal antibodies isolated by binding to the surface of live primary breast cancer cells recognize GRP78. Cancer Res 2007; 67 (19): 9507–9517.
25. Zhang Y, Liu R, Ni M et al. Cell surface relocalization of the endoplasmic reticulum chaperone and unfolded protein response regulator GRP78/BiP. J Biol Chem 2010; 285 (20): 15065–15075. doi: 10.1074/jbc.M109.087445.
26. Decca MB, Carpio MA, Bosc C et al. Post-translational arginylation of calreticulin: a new isospecies of calreticulin component of stress granules. J Biol Chem 2007; 282 (11): 8237–8245.
27. Ling S, Cheng A, Pumpens P et al. Identification of the rheumatoid arthritis shared epitope binding site on calreticulin. PLoS One 2010; 5 (7): e11703. doi: 10.1371/journal.pone.0011703.
28. Ling S, Pi X, Holoshitz J. The rheumatoid arthritis shared epitope triggers innate immune signaling via cell surface calreticulin. J Immunol 2007; 179 (9): 6359–6367.
29. Graner MW, Lillehei KO, Katsanis E. Endoplasmic reticulum chaperones and their roles in the immunogenicity of cancer vaccines. Front Oncol 2014; 4: 379. doi: 10.3389/fonc.2014.00379.
30. Eggleton P, Reid KB, Kishore U et al. Clinical relevance of calreticulin in systemic lupus erythematosus. Lupus 1997; 6 (7): 564–571.
31. Kishore U, Sontheimer RD, Sastry KN et al. The systemic lupus erythematosus (SLE) disease autoantigen-calreticulin can inhibit C1q association with immune complexes. Clin Exp Immunol 1997; 108 (2): 181–190.
32. Panaretakis T, Joza N, Modjtahedi N et al. The co-translocation of ERp57 and calreticulin determines the immunogenicity of cell death. Cell Death Differ 2008; 15 (9): 1499–1509. doi: 10.1038/cdd.2008.67.
33. Tarr JM, Winyard PG, Ryan B et al. Extracellular calreticulin is present in the joints of patients with rheumatoid arthritis and inhibits FasL (CD95L) -mediated apoptosis of T cells. Arthritis Rheum 2010; 62 (10): 2919–2929. doi: 10.1002/art.27602.
34. Weber CK, Haslbeck M, Englbrecht M et al. Antibodies to the endoplasmic reticulum-resident chaperones calnexin, BiP and Grp94 in patients with rheumatoid arthritis and systemic lupus erythematosus. Rheumatology (Oxford) 2010; 49 (12): 2255–2263. doi: 10.1093/rheumatology/keq272.
35. Tamura Y, Hirohashi Y, Kutomi G et al. Tumor-produced secreted form of binding of immunoglobulin protein elicits antigen-specific tumor immunity. J Immunol 2011; 186 (7): 4325–4330. doi: 10.4049/jimmunol.1004048.
36. Misra UK, Deedwania R, Pizzo SV. Binding of activated alpha2-macroglobulin to its cell surface receptor GRP78 in 1-LN prostate cancer cells regulates PAK-2-dependent activation of LIMK. J Biol Chem 2005; 280 (28): 26278–26286.
37. Misra UK, Deedwania R, Pizzo SV. Activation and cross- -talk between Akt, NF-kappaB, and unfolded protein response signaling in 1-LN prostate cancer cells consequent to ligation of cell surface-associated GRP78. J Biol Chem 2006; 281 (19): 13694–13707.
38. Misra UK, Gonzalez-Gronow M, Gawdi G et al. A novel receptor function for the heat shock protein Grp78: silencing of Grp78 gene expression attenuates alpha2M*-induced signalling. Cell Signal 2004; 16 (8): 929–938.
39. Liu R, Li X, Gao W et al. Monoclonal antibody against cell surface GRP78 as a novel agent in suppressing PI3K/AKT signaling, tumor growth, and metastasis. Clin Cancer Res 2013; 19 (24): 6802–6811. doi: 10.1158/1078-0432.CCR-13-1106.
40. Misra UK, Gonzalez-Gronow M, Gawdi G et al. The role of Grp 78 in alpha 2-macroglobulin-induced signal transduction. Evidence from RNA interference that the low density lipoprotein receptor-related protein is associated with, but not necessary for, GRP 78-mediated signal transduction. J Biol Chem 2002; 277 (44): 42082–42087.
41. Misra UK, Pizzo SV. Receptor-recognized alpha (2) -macroglobulin binds to cell surface-associated GRP78 and activates mTORC1 and mTORC2 signaling in prostate cancer cells. PLoS One 2012; 7 (12): e51735. doi: 10.1371/journal.pone.0051735.
42. Zhang Y, Tseng CC, Tsai YL et al. Cancer cells resistant to therapy promote cell surface relocalization of GRP78 which complexes with PI3K and enhances PI (3,4,5) P3 production. PLoS One 2013; 8 (11): e80071. doi: 10.1371/journal.pone.0080071.
43. Gonzalez-Gronow M, Cuchacovich M, Llanos C et al. Prostate cancer cell proliferation in vitro is modulated by antibodies against glucose-regulated protein 78 isolated from patient serum. Cancer Res 2006; 66 (23): 11424–12431.
44. Tsunemi S, Nakanishi T, FujitaY et al. Proteomics-based identification of a tumor-associated antigen and its corresponding autoantibody in gastric cancer. Oncol Rep 2010; 23 (4): 949–956.
45. Fu Y, Lee AS. Glucose regulated proteins in cancer progression, drug resistance and immunotherapy. Cancer Biol Ther 2006; 5 (7): 741–744.
46. Altmeyer A, Maki RG, Feldweg AM et al. Tumor-specific cell surface expression of the-KDEL containing, endoplasmic reticular heat shock protein gp96. Int J Cancer 1996; 69 (4): 340–349.
47. Bruneau N, Lombardo D, Bendayan M. Participation of GRP94-related protein in secretion of pancreatic bile salt-dependent lipase and in its internalization by the intestinal epithelium. J Cell Sci 1998; 111 (Pt 17): 2665–2679.
48. Frasson M, Vitadello M, Brunati AM et al. Grp94 is Tyr-phosphorylated by Fyn in the lumen of the endoplasmic reticulum and translocates to Golgi in differentiating myoblasts. Biochim Biophys Acta 2009; 1793 (2): 239–252. doi: 10.1016/j.bbamcr.2008.10.001.
49. Zheng H, Dai J, Stoilova D et al. Cell surface targeting of heat shock protein gp96 induces dendritic cell maturation and antitumor immunity. J Immunol 2001; 167 (12): 6731–6735.
50. Orr AW, Elzie CA, Kucik DF et al. Thrombospondin signaling through the calreticulin/LDL receptor-related protein co-complex stimulates random and directed cell migration. J Cell Sci 2003; 116 (Pt 14): 2917–2927.
51. Orr AW, Pedraza CE, Pallero MA et al. Low density lipoprotein receptor-related protein is a calreticulin coreceptor that signals focal adhesion disassembly. J Cell Biol 2003; 161 (6): 1179–1189.
52. Eggleton P, Lieu TS, Zappi EG et al. Calreticulin is released from activated neutrophils and binds to C1q and mannan-binding protein. Clin Immunol Immunopathol 1994; 72 (3): 405–409.
53. Ogden CA, deCathelineau A, Hoffmann PR et al. C1q and mannose binding lectin engagement of cell surface calreticulin and CD91 initiates macropinocytosis and uptake of apoptotic cells. J Exp Med 2001; 194 (6): 781–795.
54. Vandivier RW, Ogden CA, Fadok VA et al. Role of surfactant proteins A, D, and C1q in the clearance of apoptotic cells in vivo and in vitro: calreticulin and CD91 as a common collectin receptor complex. J Immunol 2002; 169 (7): 3978–3986.
55. Okazaki Y, Ohno H, Takase K et al. Cell surface expression of calnexin, a molecular chaperone in the endoplasmic reticulum. J Biol Chem 2000; 275 (46): 35751–35758.
56. Nimmervoll B, Chtcheglova LA, Juhasz K et al. Cell surface localised Hsp70 is a cancer specific regulator of clathrin-independent endocytosis. FEBS Lett 2015; 589 (19 Pt B): 2747–2753. doi: 10.1016/j.febslet.2015.07.037.
57. Delneste Y, Magistrelli G, Gauchat J et al. Involvement of LOX-1 in dendritic cell-mediated antigen cross-presentation. Immunity 2002; 17 (3): 353–362.
58. Theriault JR, Mambula SS, Sawamura T et al. Extracellular HSP70 binding to surface receptors present on antigen presenting cells and endothelial/epithelial cells. FEBS Lett 2005; 579 (9): 1951–1960.
59. Sims JD, McCready J, Jay DG. Extracellular heat shock protein (Hsp) 70 and Hsp90alpha assist in matrix metalloproteinase-2 activation and breast cancer cell migration and invasion. PLoS One 2011; 6 (4): e18848. doi: 10.1371/journal.pone.0018848.
60. Ellerman JE, Brown CK, de Vera M et al. Masquerader: high mobility group box-1 and cancer. Clin Cancer Res 2007; 13 (10): 2836–2848.
61. Hung CY, Tsai MC, Wu YP et al. Identification of heat-shock protein 90 beta in Japanese encephalitis virus-induced secretion proteins. J Gen Virol 2011; 92 (Pt 12): 2803–2809. doi: 10.1099/vir.0.033993-0.
62. Chen JS, Hsu YM, Chen CC et al. Secreted heat shock protein 90alpha induces colorectal cancer cell invasion through CD91/LRP-1 and NF-kappaB-mediated integrin alphaV expression. J Biol Chem 2010; 285 (33): 25458–25466. doi: 10.1074/jbc.M110.139 345.
63. Li W, Li Y, Guan S et al. Extracellular heat shock protein-90alpha: linking hypoxia to skin cell motility and wound healing. EMBO J 2007; 26 (5): 1221–1233.
64. Liao DF, Jin ZG, Baas AS et al. Purification and identification of secreted oxidative stress-induced factors from vascular smooth muscle cells. J Biol Chem 2000; 275 (1): 189–196.
65. Yu X, Harris SL, Levine AJ. The regulation of exosome secretion: a novel function of the p53 protein. Cancer Res 2006; 66 (9): 4795–4801.
66. Li W, Tsen F, Sahu D et al. Extracellular Hsp90 (eHsp90) as the actual target in clinical trials: intentionally or unintentionally. Int Rev Cell Mol Biol 2013; 303: 203–235. doi: 10.1016/B978-0-12-407697-6.00005-2.
67. McCready J, Sims JD, Chan D et al. Secretion of extracellular hsp90alpha via exosomes increases cancer cell motility: a role for plasminogen activation. BMC Cancer 2010; 10: 294. doi: 10.1186/1471-2407-10-294.
68. Eustace BK, Jay DJ. Extracellular roles for the molecular chaperone, hsp90. Cell Cycle 2004; 3 (9): 1098–1100.
69. Colaco C. Autologous heat-shock protein vaccines. Hum Vaccin Immunother 2013; 9 (2): 275–276.
70. Randazzo M, Terness P, Opelz G et al. Active-specific immunotherapy of human cancers with the heat shock protein Gp96-revisited. Int J Cancer 2012; 130 (10): 2219–2231. doi: 10.1002/ijc.27332.
71. Reitsma DJ, Combest AJ. Challenges in the development of an autologous heat shock protein based anti-tumor vaccine. Hum Vaccin Immunother 2012; 8 (8): 1152–1155. doi: 10.4161/hv.21382.
72. Gao P, Sun X, Chen X et al. Secretion of stress protein grp170 promotes immune-mediated inhibition of murine prostate tumor. Cancer Immunol Immunother 2009; 58 (8): 1319–1328. doi: 10.1007/s00262-008-0647-6.
73. Yu X, Guo C, Yi H et al. A multifunctional chimeric chaperone serves as a novel immune modulator inducing therapeutic antitumor immunity. Cancer Res 2013; 73 (7): 2093–2103. doi: 10.1158/0008-5472.CAN-12-1740.
Štítky
Paediatric clinical oncology Surgery Clinical oncologyČlánok vyšiel v časopise
Clinical Oncology
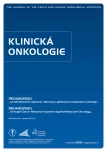
2016 Číslo Supplementum 4
- Spasmolytic Effect of Metamizole
- Metamizole at a Glance and in Practice – Effective Non-Opioid Analgesic for All Ages
- Metamizole in perioperative treatment in children under 14 years – results of a questionnaire survey from practice
- Current Insights into the Antispasmodic and Analgesic Effects of Metamizole on the Gastrointestinal Tract
- Obstacle Called Vasospasm: Which Solution Is Most Effective in Microsurgery and How to Pharmacologically Assist It?
Najčítanejšie v tomto čísle
- The Role of PD-1/PD-L1 Signaling Pathway in Antitumor Immune Response
- Non-Small Cell Lung Cancer – from Immunobiology to Immunotherapy
- Cancer Cells as Dynamic System – Molecular and Phenotypic Changes During Tumor Formation, Progression and Dissemination
- Novel Approaches in DNA Methylation Studies – MS-HRM Analysis and Electrochemistry