Mechanisms of Protein Homeostasis Regulation in Cancer Development
Authors:
F. Trčka; P. Müller; B. Vojtěšek
Authors place of work:
Regionální centrum aplikované molekulární onkologie, Masarykův onkologický ústav, Brno
Published in the journal:
Klin Onkol 2016; 29(Supplementum 4): 18-24
Category:
Review
doi:
https://doi.org/10.14735/amko20164S18
Summary
Background:
The proteome of eukaryotic cells represents a complex system. Its components are exposed to various intrinsic and extrinsic stresses. Therefore, the function of the cellular proteome is dependent on the existence of compensatory mechanisms balancing the inner protein homeostasis – proteostasis. These mechanisms involve the network of molecular chaperones and transcriptional program regulating their expression. The process of cancerogenesis is accompanied by significant changes in the intracellular milieu of cancer cells – temperature, pH, availability of nutrients. On the one hand, these changes represent a consequence of the deregulated growth of the tumor tissue; on the other hand, they can be a source of selection pressure, which allows the emergence of resistant and aggressive tumor cell populations. Description of the proteostatic apparatus components and the mechanism of their involvement in the tumor tissue development is provided in this review article.
Aim:
This review focuses on the description of two causally linked groups of proteostatic events; their mutual coordination is crucial to the process of tumor cell and by extension the entire tumor tissue response to environmental and internal stress factors. The first group of these processes is represented by the “executory” role of molecular chaperones from HSP70, HSP90 and so-called small molecular chaperone protein families. These proteins are involved in maintaining stability of cellular proteins essential for proliferation, apoptosis, senescence, migration and phenotypic plasticity of tumor cells. The second group of the described processes comprises the posttranslational control of the “systemic” role of the transcription factor HSF1 in regulating the gene expression of molecular chaperones and other genes specifically regulated by this transcription factor in the tumor and stromal cells.
Key words:
molecular chaperones – heat-shock factor 1 – cancer – protein homeostasis
This work was supported by the project MEYS – NPS I – LO1413.
The authors declare they have no potential conflicts of interest concerning drugs, products, or services used in the study.
The Editorial Board declares that the manuscript met the ICMJE recommendation for biomedical papers.
Submitted:
15. 5. 2016
Accepted:
25. 5. 2016
Zdroje
1. Labbadia J, Morimoto RI. The biology of proteostasis in aging and disease. Annu Rev Biochem 2015; 84: 435–464. doi: 10.1146/annurev-biochem-060614-033955.
2. Trcka F, Vojtesek B, Muller P. Protein quality control and cancerogenesis. Klin Onkol 2012; 25 (Suppl 2): 2S38–2S44.
3. Tang Z, Dai S, He Y et al. MEK guards proteome stability and inhibits tumor-suppressive amyloidogenesis via HSF1. Cell 2015; 160 (4): 729–744. doi: 10.1016/ j.cell.2015.01.028.
4. Morimoto RI. The heat shock response: systems biology of proteotoxic stress in aging and disease. Cold Spring Harb Symp Quant Biol 2011; 76: 91–99. doi: 10.1101/sqb.2012.76.010637.
5. Anckar J, Sistonen L. Regulation of HSF1 function in the heat stress response: implications in aging and disease. Annu Rev Biochem 2011; 80: 1089–1115. doi: 10.1146/annurev-biochem-060809-095203.
6. Vihervaara A, Sistonen L. HSF1 at a glance. J Cell Sci 2014; 127 (Pt 2): 261–266. doi: 10.1242/jcs.132605.
7. Zhang Y, Huang L, Zhang J et al. Targeted disruption of hsf1 leads to lack of thermotolerance and defines tissue-specific regulation for stress-inducible Hsp molecular chaperones. J Cell Biochem 2002; 86 (2): 376–393.
8. Izu H, Inouye S, Fujimoto M et al. Heat shock transcription factor 1 is involved in quality-control mechanisms in male germ cells. Biol Reprod 2004; 70 (1): 18–24.
9. Ruggero D, Pandolfi PP. Does the ribosome translate cancer? Nat Rev Cancer 2003; 3 (3): 179–192.
10. Roux PP, Topisirovic I. Regulation of mRNA translation by signaling pathways. Cold Spring Harb Perspect Biol 2012; 4 (11): pii: a012252. doi: 10.1101/cshperspect.a012252.
11. Santagata S, Mendillo ML, Tang YC et al. Tight coordination of protein translation and HSF1 activation supports the anabolic malignant state. Science 2013; 341 (6143): 1238303. doi: 10.1126/science.1238303.
12. Dai C, Santagata S, Tang Z et al. Loss of tumor suppressor NF1 activates HSF1 to promote carcinogenesis. J Clin Invest 2012; 122 (10): 3742–3754. doi: 10.1172/JCI62727.
13. Ciocca DR, Arrigo AP, Calderwood SK. Heat shock proteins and heat shock factor 1 in carcinogenesis and tumor development: an update. Arch Toxicol 2013; 87 (1): 19–48. doi: 10.1007/s00204-012-0918-z.
14. Hunt C, Morimoto RI. Conserved features of eukaryotic hsp70 genes revealed by comparison with the nucleotide sequence of human hsp70. Proc Natl Acad Sci U S A 1985; 82 (19): 6455–6459.
15. Chen B, Zhong D, Monteiro A. Comparative genomics and evolution of the HSP90 family of genes across all kingdoms of organisms. BMC Genomics 2006; 7: 156.
16. Sreedhar AS, Kalmár E, Csermely P et al. Hsp90 isoforms: functions, expression and clinical importance. FEBS Lett 2004; 562 (1–3): 11–15.
17. Young JC, Hoogenraad NJ, Hartl FU. Molecular chaperones Hsp90 and Hsp70 deliver preproteins to the mitochondrial import receptor Tom70. Cell 2003; 112 (1): 41–50.
18. Mayer MP, Schroder H, Rudiger S et al. Multistep mechanism of substrate binding determines chaperone activity of Hsp70. Nat Struct Biol 2000; 7 (7): 586–593.
19. Li J, Buchner J. Structure, function and regulation of the hsp90 machinery. Biomed J 2013; 36 (3): 106–117. doi: 10.4103/2319-4170.113230.
20. Mayer MP, Bukau B. Hsp70 chaperones: cellular functions and molecular mechanism. Cell Mol Life Sci 2005; 62 (6): 670–684.
21. Taipale M, Tucker G, Peng J et al. A quantitative chaperone interaction network reveals the architecture of cellular protein homeostasis pathways. Cell 2014; 158 (2): 434–448. doi: 10.1016/j.cell.2014.05.039.
22. Taipale M, Krykbaeva I, Koeva M et al. Quantitative analysis of HSP90-client interactions reveals principles of substrate recognition. Cell 2012; 150 (5): 987–1001. doi: 10.1016/j.cell.2012.06.047.
23. Kirschke E, Goswami D, Southworth D et al. Glucocorticoid receptor function regulated by coordinated action of the Hsp90 and Hsp70 chaperone cycles. Cell 2014; 157 (7): 1685–1697. doi: 10.1016/j.cell.2014.04. 038.
24. Calderwood SK. Molecular cochaperones: tumor growth and cancer treatment. Scientifica (Cairo) 2013; 2013: ID 217513. doi: 10.1155/2013/217513.
25. Haslbeck M, Franzmann T, Weinfurtner D et al. Some like it hot: the structure and function of small heat-shock proteins. Nat Struct Mol Biol 2005; 12 (10): 842–846.
26. Cashikar AG, Duennwald M, Lindquist SL. A chaperone pathway in protein disaggregation. Hsp26 alters the nature of protein aggregates to facilitate reactivation by Hsp104. J Biol Chem 2005; 280 (25): 23869–23875.
27. van Montfort RL, Basha E, Friedrich KL et al. Crystal structure and assembly of a eukaryotic small heat shock protein. Nat Struct Biol 2001; 8 (12): 1025–1030.
28. Arrigo AP, Simon S, Gibert B et al. Hsp27 (HspB1) and alphaB-crystallin (HspB5) as therapeutic targets. FEBS Lett 2007; 581 (19): 3665–3674.
29. Hanahan D, Weinberg RA. Hallmarks of cancer: the next generation. Cell 2011; 144 (5): 646–674. doi: 10.1016/j.cell.2011.02.013.
30. Momeny M, Saunus JM, Marturana F et al. Heregulin-HER3-HER2 signaling promotes matrix metalloproteinase-dependent blood-brain-barrier transendothelial migration of human breast cancer cell lines. Oncotarget 2015; 6 (6): 3932–3946.
31. Hobbs GA, Der CJ, Rossman KL. RAS isoforms and mutations in cancer at a glance. J Cell Sci 2016; 129 (7): 1287–1292. doi: 10.1242/jcs.182873.
32. Wang S, Cang S, Liu D. Third-generation inhibitors targeting EGFR T790M mutation in advanced non-small cell lung cancer. J Hematol Oncol 2016; 9: 34. doi: 10.1186/s13045-016-0268-z.
33. Joab I, Radanyi C, Renoir M et al. Common non-hormone binding component in non-transformed chick oviduct receptors of four steroid hormones. Nature 1984; 308 (5962): 850–853.
34. Sanchez ER, Toft DO, Schlesinger MJ et al. Evidence that the 90-kDa phosphoprotein associated with the untransformed L-cell glucocorticoid receptor is a murine heat shock protein. J Biol Chem 1985; 260 (23): 12398–12401.
35. Zhang X, Gureasko J, Shen K et al. An allosteric mechanism for activation of the kinase domain of epidermal growth factor receptor. Cell 2006; 125 (6): 1137–1149.
36. Wan PT, Garnett MJ, Roe SM et al. Mechanism of activation of the RAF-ERK signaling pathway by oncogenic mutations of B-RAF. Cell 2004; 116 (6): 855–867.
37. Shimamura T, Lowell AM, Engelman JA et al. Epidermal growth factor receptors harboring kinase domain mutations associate with the heat shock protein 90 chaperone and are destabilized following exposure to geldanamycins. Cancer Res 2005; 65 (14): 6401–6408.
38. da Rocha Dias S, Friedlos F, Light Y et al. Activated B-RAF is an Hsp90 client protein that is targeted by the anticancer drug 17-allylamino-17-demethoxygeldanamycin. Cancer Res 2005; 65 (23): 10686–10691.
39. Tao W, Chakraborty SN, Leng X et al. HSP90 inhibitor AUY922 induces cell death by disruption of the Bcr-Abl, Jak2 and HSP90 signaling network complex in leukemia cells. Genes Cancer 2015; 6 (1–2): 19–29.
40. Brugge JS, Erikson E, Erikson RL. The specific interaction of the Rous sarcoma virus transforming protein, pp60src, with two cellular proteins. Cell 1981; 25 (2): 363–372.
41. Lipsich LA, Cutt JR, Brugge JS. Association of the transforming proteins of Rous, Fujinami, and Y73 avian sarcoma viruses with the same two cellular proteins. Mol Cell Biol 1982; 2 (7): 875–880.
42. Vaughan CK, Gohlke U, Sobott F et al. Structure of an Hsp90-Cdc37-Cdk4 complex. Mol Cell 2006; 23 (5): 697–707.
43. Scholz GM, Hartson SD, Cartledge K et al. The molecular chaperone Hsp90 is required for signal transduction by wild-type Hck and maintenance of its constitutively active counterpart. Cell Growth Differ 2001; 12 (8): 409–417.
44. Xu W, Yuan X, Xiang Z et al. Surface charge and hydrophobicity determine ErbB2 binding to the Hsp90 chaperone complex. Nat Struct Mol Biol 2005; 12 (2): 120–126.
45. Meng L, Hunt C, Yaglom JA et al. Heat shock protein Hsp72 plays an essential role in Her2-induced mammary tumorigenesis. Oncogene 2011; 30 (25): 2836–2845. doi: 10.1038/onc.2011.5.
46. Gong J, Weng D, Eguchi T et al. Targeting the hsp70 gene delays mammary tumor initiation and inhibits tumor cell metastasis. Oncogene 2015; 34 (43): 5460–5471. doi: 10.1038/onc.2015.1.
47. Ouyang L, Shi Z, Zhao S et al. Programmed cell death pathways in cancer: a review of apoptosis, autophagy and programmed necrosis. Cell Prolif 2012; 45 (6): 487–498. doi: 10.1111/j.1365-2184.2012.00845.x.
48. Lanneau D, de Thonel A, Maurel S et al. Apoptosis versus cell differentiation: role of heat shock proteins HSP90, HSP70 and HSP27. Prion 2007; 1 (1): 53–60.
49. Chauhan D, Li G, Hideshima T et al. Hsp27 inhibits release of mitochondrial protein Smac in multiple myeloma cells and confers dexamethasone resistance. Blood 2003; 102 (9): 3379–3386.
50. Paul C, Simon S, Gibert B et al. Dynamic processes that reflect anti-apoptotic strategies set up by HspB1 (Hsp27). Exp Cell Res 2010; 316 (9): 1535–1552. doi: 10.1016/j.yexcr.2010.03.006.
51. Zenke G, Strittmatter U, Tees R et al. A cocktail of three monoclonal antibodies significantly increases the sensitivity of an enzyme immunoassay for human granulocyte-macrophage colony-stimulating factor. J Immunoassay 1991; 12 (2): 185–206.
52. Arrigo AP, Gibert B. HspB1 dynamic phospho-oligomeric structure dependent interactome as cancer therapeutic target. Curr Mol Med 2012; 12 (9): 1151–1163.
53. Beere HM, Wolf BB, Cain K et al. Heat-shock protein 70 inhibits apoptosis by preventing recruitment of procaspase-9 to the Apaf-1 apoptosome. Nat Cell Biol 2000; 2 (8): 469–475.
54. Jaskelioff M, Song W, Xia J et al. Telomerase deficiency and telomere dysfunction inhibit mammary tumors induced by polyomavirus middle T oncogene. Oncogene 2009; 28 (48): 4225–4236. doi: 10.1038/onc.2009.268.
55. Toogun OA, Dezwaan DC, Freeman BC. The hsp90 molecular chaperone modulates multiple telomerase activities. Mol Cell Biol 2008; 28 (1): 457–467.
56. Chaklader M, Das P, Pereira JA et al. 17-AAG mediated targeting of Hsp90 limits tert activity in peritoneal sarcoma related malignant ascites by downregulating cyc- lin D1 during cell cycle entry. Exp Oncol 2012; 34 (2): 90–96.
57. Kim RH, Kim R, Chen W et al. Association of hsp90 to the hTERT promoter is necessary for hTERT expression in human oral cancer cells. Carcinogenesis 2008; 29 (12): 2425–2431. doi: 10.1093/carcin/bgn225.
58. Lipsyc M, Yaeger R. Impact of somatic mutations on patterns of metastasis in colorectal cancer. J Gastrointest Oncol 2015; 6 (6): 645–649. doi: 10.3978/j.issn.2078-6891.2015.045.
59. Dhamija S, Diederichs S. From junk to master regulators of invasion: lncRNA functions in migration, EMT and metastasis. Int J Cancer 2016; 139 (2): 269–280. doi: 10.1002/ijc.30039.
60. Tsutsumi S, Beebe K, Neckers L. Impact of heat-shock protein 90 on cancer metastasis. Future Oncol 2009; 5 (5): 679–688. doi: 10.2217/fon.09.30.
61. Pavan S, Musiani D, Torchiaro E et al. HSP27 is required for invasion and metastasis triggered by hepatocyte growth factor. Int J Cancer 2014; 134 (6): 1289–1299. doi: 10.1002/ijc.28464.
62. Shiota M, Bishop JL, Nip KM et al. Hsp27 regulates epithelial mesenchymal transition, metastasis, and circulating tumor cells in prostate cancer. Cancer Res 2013; 73 (10): 3109–3119.
63. Gibert B, Eckel B, Gonin V et al. Targeting heat shock protein 27 (HspB1) interferes with bone metastasis and tumour formation in vivo. Br J Cancer 2012; 107 (1): 63–70. doi: 10.1038/bjc.2012.188.
64. Rutherford SL, Lindquist S. Hsp90 as a capacitor for morphological evolution. Nature 1998; 396 (6709): 336–342.
65. Burrell RA, McGranahan N, Bartek J et al. The causes and consequences of genetic heterogeneity in cancer evolution. Nature 2013; 501 (7467): 338–345. doi: 10.1038/nature12625.
66. Turtoi A, Blomme A, Castronovo V. Intratumoral heterogeneity and consequences for targeted ther- apies. Bull Cancer 2015; 102 (1): 17–23. doi: 10.1016/j.bulcan.2014.12.006.
67. Shi Y, Mosser DD, Morimoto RI. Molecular chaperones as HSF1-specific transcriptional repressors. Genes Dev 1998; 12 (5): 654–666.
68. Ali A, Bharadwaj S, O‘Carroll R et al. HSP90 interacts with and regulates the activity of heat shock factor 1 in Xenopus oocytes. Mol Cell Biol 1998; 18 (9): 4949–4960.
69. Akerfelt M, Morimoto RI, Sistonen L. Heat shock factors: integrators of cell stress, development and lifespan. Nat Rev Mol Cell Biol 2010; 11 (8): 545–555. doi: 10.1038/nrm2938.
70. Raychaudhuri S, Loew C, Korner R et al. Interplay of acetyltransferase EP300 and the proteasome system in regulating heat shock transcription factor 1. Cell 2014; 156 (5): 975–985. doi: 10.1016/j.cell.2014.01.055.
71. Brunet Simioni M, De Thonel A, Hammann A et al. Heat shock protein 27 is involved in SUMO-2/3 modification of heat shock factor 1 and thereby modulates the transcription factor activity. Oncogene 2009; 28 (37): 3332–3344. doi: 10.1038/onc.2009.188.
72. Holmberg CI, Hietakangas V, Mikhailov A et al. Phosphorylation of serine 230 promotes inducible transcriptional activity of heat shock factor 1. EMBO J 2001; 20 (14): 3800–3810.
73. Zhang Y, Murshid A, Prince T et al. Protein kinase A regulates molecular chaperone transcription and protein aggregation. PLoS One 2011; 6 (12): e28950. doi: 10.1371/journal.pone.0028950.
74. Soncin F, Zhang X, Chu B et al. Transcriptional activity and DNA binding of heat shock factor-1 involve phosphorylation on threonine 142 by CK2. Biochem Biophys Res Commun 2003; 303 (2): 700–706.
75. Kim SA, Yoon JH, Lee SH et al. Polo-like kinase 1 phosphorylates heat shock transcription factor 1 and mediates its nuclear translocation during heat stress. J Biol Chem 2005; 280 (13): 12653–12657.
76. Guettouche T, Boellmann F, Lane WS et al. Analysis of phosphorylation of human heat shock factor 1 in cells experiencing a stress. BMC Biochem 2005; 6: 4.
77. Chou SD, Prince T, Gong J et al. mTOR is essential for the proteotoxic stress response, HSF1 activation and heat shock protein synthesis. PLoS One 2012; 7 (6): e39679. doi: 10.1371/journal.pone.0039679.
78. Samatar AA, Poulikakos PI. Targeting RAS-ERK signalling in cancer: promises and challenges. Nat Rev Drug Discov 2014; 13 (12): 928–942. doi: 10.1038/nrd4 281.
79. Fernández-Medarde A, Santos E. Ras in cancer and developmental diseases. Genes Cancer 2011; 2 (3): 344–358. doi: 10.1177/1947601911411084.
80. Dai S, Tang Z, Cao J et al. Suppression of the HSF1-mediated proteotoxic stress response by the metabolic stress sensor AMPK. EMBO J 2015; 34 (3): 275–293. doi: 10.15252/embj.201489062.
81. Hardie DG. AMPK: positive and negative regulation, and its role in whole-body energy homeostasis. Curr Opin Cell Biol 2015; 33: 1–7. doi: 10.1016/j.ceb.2014.09.004.
82. Zoncu R, Efeyan A, Sabatini DM. mTOR: from growth signal integration to cancer, diabetes and ageing. Nat Rev Mol Cell Biol 2011; 12 (1): 21–35. doi: 10.1038/nrm 3025.
83. Noda T, Ohsumi Y. Tor, a phosphatidylinositol kinase homologue, controls autophagy in yeast. J Biol Chem 1998; 273 (7): 3963–3966.
84. Acquaviva J, He S, Sang J et al. mTOR inhibition potentiates HSP90 inhibitor activity via cessation of HSP synthesis. Mol Cancer Res 2014; 12 (5): 703–713. doi: 10.1158/1541-7786.MCR-13-0605.
85. Showkat M, Beigh MA, Andrabi KI. mTOR signaling in protein translation regulation: implications in cancer genesis and therapeutic interventions. Mol Biol Int 2014; 2014: ID 686984. doi: 10.1155/2014/686984.
86. Kaitsuka T, Tomizawa K, Matsushita M. Transformation of eEF1Bdelta into heat-shock response transcription factor by alternative splicing. EMBO Rep 2011; 12 (7): 673–681. doi: 10.1038/embor.2011.82.
87. Brandman O, Stewart-Ornstein J, Wong D et al. A ribosome-bound quality control complex triggers degradation of nascent peptides and signals translation stress. Cell 2012; 151 (5): 1042–1054. doi: 10.1016/j.cell.2012.10.044.
88. Brandman O, Hegde RS. Ribosome-associated protein quality control. Nat Struct Mol Biol 2016; 23 (1): 7–15.
89. Shen PS, Park J, Qin Y et al. Protein synthesis. Rqc2p and 60S ribosomal subunits mediate mRNA-independent elongation of nascent chains. Science 2015; 347 (6217): 75–78. doi: 10.1126/science.1259724.
90. Trinklein ND, Murray JI, Hartman SJ et al. The role of heat shock transcription factor 1 in the genome-wide regulation of the mammalian heat shock response. Mol Biol Cell 2004; 15 (3): 1254–1261.
91. Mendillo ML, Santagata S, Koeva M et al. HSF1 drives a transcriptional program distinct from heat shock to support highly malignant human cancers. Cell 2012; 150 (3): 549–562. doi: 10.1016/j.cell.2012.06. 031.
92. Scherz-Shouval R, Santagata S, Mendillo ML et al. The reprogramming of tumor stroma by HSF1 is a potent enabler of malignancy. Cell 2014; 158 (3): 564–578. doi: 10.1016/j.cell.2014.05.045.
93. Douglas PM, Baird NA, Simic MS et al. Heterotypic signals from neural HSF-1 separate thermotolerance from longevity. Cell Rep 2015; 12 (7): 1196–1204.
Štítky
Paediatric clinical oncology Surgery Clinical oncologyČlánok vyšiel v časopise
Clinical Oncology
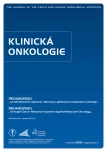
2016 Číslo Supplementum 4
- Spasmolytic Effect of Metamizole
- Metamizole at a Glance and in Practice – Effective Non-Opioid Analgesic for All Ages
- Metamizole in perioperative treatment in children under 14 years – results of a questionnaire survey from practice
- Current Insights into the Antispasmodic and Analgesic Effects of Metamizole on the Gastrointestinal Tract
- Obstacle Called Vasospasm: Which Solution Is Most Effective in Microsurgery and How to Pharmacologically Assist It?
Najčítanejšie v tomto čísle
- The Role of PD-1/PD-L1 Signaling Pathway in Antitumor Immune Response
- Non-Small Cell Lung Cancer – from Immunobiology to Immunotherapy
- Cancer Cells as Dynamic System – Molecular and Phenotypic Changes During Tumor Formation, Progression and Dissemination
- Novel Approaches in DNA Methylation Studies – MS-HRM Analysis and Electrochemistry