Buccal Epithelial Cells as Potential Non‑ invasive Materials for the Monitoring of Mitochondrial Disturbances to Track Huntington‘s Disease Progression – a Pilot Study
Epiteliální buňky bukálního stěru jako potenciální neinvazivní biologický materiál pro monitorování mitochondriálních dysfunkcí v průběhu rozvoje Huntingtonovy choroby – pilotní studie
Úvod:
Huntingtonova nemoc (Huntington’s Disease; HD) je neléčitelné neurodegenerativní onemocnění, které se manifestuje v dospělosti progresivními změnami chování, motoriky a kognitivních funkcí. Onemocnění je způsobeno expanzí tripletu CAG v genu pro protein huntingtin a má téměř úplnou penetranci. Nejvíce postižené bývá striatum, avšak patologické změny jsou detekovatelné také v periferních tkáních jako je krev, sval nebo kožní fibroblasty. V posledních letech se ukazuje, že důležitou roli v patogenezi a progresi HD hraje poškození mitochondrií, jako jsou např. změny aktivit komplexů dýchacího řetězce, snížení syntézy ATP nebo zvýšení apoptotických signálů.
Cíl a materiál:
Cílem studie bylo analyzovat množství komplexu I a IV dýchacího řetězce v epiteliálních buňkách bukálního stěru u šesti pacientů s HD a u tří miniprasat transgenních pro N‑terminální část lidského mutovaného huntingtinu (TgHD) ve srovnání se zdravými kontrolami. Metody: Množství komplexu I a IV dýchacího řetězce bylo stanoveno pomocí mikroimunoanalýzy (Dipstick microimmunocapture assay, Mitosciences).
Výsledky:
U čtyř pacientů ze šesti bylo detekováno snížené množství komplexu I a/ nebo IV až na 36 % hodnoty u kontrol. Mikroimunoanalýza se ukázala dostatečně citlivá i pro detekci komplexů I a IV v buňkách bukální sliznice TgHD miniprasat, avšak ve věku 36 měsíců nebyl zaznamenán rozdíl v obsahu komplexů I a IV mezi TgHD a WT zvířaty. Vzorky z bukálního stěru mohou být odebírány opakovaně bez větší zátěže pacienta nebo sledovaného zvířete. Analýza epiteliálních buněk ústní sliznice tak může sloužit k dlouhodobému monitorování mitochondrií od asymptomatického období až po úplný rozvoj HD.
Klíčová slova:
Huntingtonova nemoc – komplex I dýchacího řetězce – komplex IV dýchacího řetězce – epiteliální buňky bukálního stěru
Autoři deklarují, že v souvislosti s předmětem studie nemají žádné komerční zájmy.
Redakční rada potvrzuje, že rukopis práce splnil ICMJE kritéria pro publikace zasílané do biomedicínských časopisů.
Authors:
M. Rodinova 1; E. Trefilova 1; I. Liskova 2,3; J. Klempir 2,4; Z. Ellederová 3; D. Vidinska 3; J. Motlik 3; J. Zeman 1; H. Hansíková 1
Authors‘ workplace:
Department of Pediatrics and Adolescent Medicine, 1st Faculty of Medicine, Charles University and General University Hospital in Prague, Czech Republic
1; Department of Neurology and Centre of Clinical Neuroscience, 1st Faculty of Medicine, Charles University and General University Hospital in Prague, Czech Republic
2; Laboratory of Cell Regeneration and Cell Plasticity, Institute of Animal Physiology and Genetics AS CR, v. v. i., Libechov, Czech Republic
3; Institute of Anatomy, 1st Faculty of Medicine, Charles University in Prague, Czech Republic
4
Published in:
Cesk Slov Neurol N 2015; 78/111(Supplementum 2): 49-54
doi:
https://doi.org/10.14735/amcsnn2012S49
Overview
Introduction:
Huntington’s disease (HD) is an incurable neurodegenerative disorder that manifests progressive behavioral and motoric changes and cognitive dysfunction. It is caused by CAG expansion in huntingtin gene and has almost full penetrance. The striatum is the most affected tissue in HD, but pathological changes are also detected in the peripheral tissues like blood, skeletal muscle or fibroblasts. Intensive HD research in the last years has revealed an important role of mitochondrial dysfunction in the pathogenesis and progression of the disease, which includes changes in the activity of the respiratory chain complexes, ATP synthesis or increased apoptotic stimuli.
Aim and material:
We decided to analyse the amount of mitochondrial respiratory chain complex I and IV in the epithelial cells of buccal smear in six HD patients and from an animal disease model, minipigs transgenic for the N‑terminal part of human mutated huntingtin (TgHD). Methods: The amount of mitochondrial complexes I and IV was determined by dipstick microimmunocapture assay (Mitosciences).
Results:
A decreased level of CI and/ or CIV in HD patients (4/ 6), up to 36% of the control values, was detected. The analysis performed on the minipig samples showed strong signals, but no uniform trend was observed within the WT and TgHD groups at the age of 36 months.
Conclusions:
The advantage of this approach is that samples of buccal cells can be collected repeatedly without the stress for the studied objects and therefore can serve for longitudinal monitoring of mitochondria during the asymptomatic stage until full development of the disease.
Key words:
Huntington’s disease – mitochondrial respiratory chain complex I and IV – buccal epithelial cells
Introduction
Huntington’s disease (HD) is an autosomal dominant neurodegenerative disorder usually affecting adult people between 30 and 50 years of age. HD is characterized by slow changes in behavior and personality, motoric changes and progressive dementia [1]. The mean duration of HD is 17– 20 years and is caused by the pathological expansion of a CAG repetition on chromosome 4 in coding area of the gene for protein huntingtin (Htt) [2]. A normal polyglutamine Htt tract contains 10– 35 glutamines; HD patients usually carry more than 40 CAG repetitions [3]. The onset and course of the disease are, to some extent, influenced by the number of repeats, where a higher CAG number means earlier onset and faster progression [4].
Htt is important for brain development, neuronal function and neuronal stability [5]. The brain, especially the striatum, is the tissue most affected by HD. Intensive research in this area has revealed new functions of Htt and connected them with a variety of cellular processes not only specific for neurons. One example of such a connection is the mitochondrial link of Htt functions and HD. It is known that Htt binds to the outer membrane of the mitochondria and impacts fusion/ fission machinery. Htt also influences the transcriptional factor Peroxisome proliferator‑activated receptor γ coactivator‑ 1α (PGC1– α), which manages mitochondrial energy metabolism in many ways, which leads to changes in whole cellular physiology [6]. The mitochondria’s connection to HD has been intensively studied, and the disease is linked to many mitochondrial disturbances, such as the decreased activity of respiratory chain complex II (CII) [7], decreased oxygen consumption [8] and ultrastructural changes in neuronal tissues [9]. Pathological disturbances in HD are not strictly connected only to neuronal tissues, and the peripheral tissues are now a focus of interest [10]. The mitochondrial pathology in the peripheral tissues has been described in muscle [10], the heart [11], lymphocytes [12] and fibroblasts [13], as well as in the testes [14]. In order to find a specific marker that could monitor the course of disease in HD, particularly in non‑invasive tissue, we decided to test the cells of buccal smear.
In our study, we determined the amount of mitochondrial respiratory chain complex I (CI) and complex IV (CIV) in buccal epithelial cells obtained from HD patients and from animal disease model, minipigs transgenic (TgHD) for the terminal part of human mutated huntingtin.
Materials and methods
Patients
The experimental group consisted of six HD patients and two healthy adult controls. In the patient group, there were three men and three women between the ages of 34 and 66 years. All patients were symptomatic, heterozygote HD patients, in which the CAG repeat number on the mutated allele varied between 42 and 53. The number of CAG repeats and the course of the disease corresponded to the classical form of HD in all cases. Informed consent was obtained from patients prior to taking samples, and the Ethical Committee of the General University Hospital in Prague approved the study. The detailed information of each patient, such as the age of disease onset and symptoms, are summarized in Tab. 1.
Animals
Transgenic minipigs with the N‑terminal part of the human mutated huntingtin [27] were studied. The transgenic (n = 3, marked K63, K100 and K104) and wild type (WT) controls (n = 3, marked K48, K64 and K103) at the age of 36 months were used in experiments. K48, K100 were females, K64, K103, K104, K63 were males. All components of this study were carried out in accordance with the Animal Care and Use Committee of Institute of Animal Physiology and Genetics and were conducted according to current Czech regulations and guidelines for animal welfare and with approval by the State Veterinary Administration of the Czech Republic.
Sample collection and analysis
Buccal epithelial cells were obtained noninvasively from patients, healthy controls, TgHD and wild type animals for this study. Buccal epithelial cells were collected using a sterile OmniSwab (Ct. No. WB100035, Whatman, Whatman/ GE Healthcare Europe GmbH, Freiburg, Germany). The OmniSwab brush was used to scrape the inside of both cheeks. The scrape was provided gently to prevent blood contamination of the sample because of injury. The cotton brush with the collected cells was transferred into a clean microtube and stored at – 80 °C until use.
The expression of Htt and mtHtt in buccal epithelial cells from samples taken from the patients and animals was verified using electrophoretic separation (Fig. 1), the visualisation of Htt and mtHtt was done by the N‑terminal unit of huntingtin antibody (EPR5526, Abcam).
Dipstick immunocapture analysis
Dipstick immunocapture analysis (DIA) kits were used to determine the level of mitochondrial CI and CIV in buccal epithelial cells(ab109722, ab109877, Complex I and IV Human Protein Quantity Dipstick Assay Kit, Abcam). All procedures were carried out according to conditions determined in our previous study [16] and the manufacturer’s instruction, with minor modifications, as mentioned briefly below. Buccal cell isolation was performed by adding 600 µl of buffer A (ab109722 or ab109877, these solutions are interchangeable) and a 1% protease inhibitory cocktail (PIC, Sigma‑ Aldrich, St. Louis, MO) to an Eppendorf tube (Eppendorf, Hamburg, Germany) containing a swab with the collected buccal epithelial cells. The tube was incubated on ice with frequent mixing (every 5 min) for 1 hour and then centrifuged at 15,000 g for 20 min at 4°C. The supernatant (the buccal cell lysate) was used for further analyses or stored at – 80 °C until use.
For the dipstick analysis, 100 µg of protein per buccal cell lysate sample was diluted into 50 µl in buffer A. A volume of 25 µl of buffer B was added to the sample, and the mixture was transferred to a microplate well containing a dried gold‑ conjugated antibody (ab109722 or ab109877). After antibody rehydration, the dipstick was added, and sample was wicked onto it. Then, 30 µl of buffer C was added to the well to wash the high background away from the dipstick. The dipstick was dried and scanned. The signal intensity of CIV/ CI was analysed using the Quantity One 1– D Analysis Software (Bio‑ Rad Laboratories, Hercules, CA). The results of two independent measurements conducted under the same conditions were evaluated separately using the Quantity One 1– D Analysis Software (Bio‑ Rad Laboratories), and the resulting scores were averaged and interpreted. The signal intensities of the patient samples were expressed in percentage units. The signal intensities of the minipig samples were expressed in absolute values.
Results
Patient testing
DIA (ab109722, ab109877, Abcam) was used to determine the amounts of CI and CIV in buccal epithelial cells obtained from six HD patients and two adult healthy controls. The results of two independent measurements obtained under the same conditions were evaluated separately using the Quantity One 1-D Analysis Software (Bio‑ Rad Laboratories), and the scores were averaged and interpreted.
The amount of CI was decreased in four patients (P3, P4, P5 and P6). The amount of CI in the buccal epithelial cells of patients P1 and P2 was at the lower limits of the control values (Fig. 2A). The amount of CI in patients P1, P2, P3, P4, P5, and P6 was 86%, 76%, 71%, 70%, 62%, and 36% of the control values, respectively.
The determination of the amount of CIV in the buccal epithelial cells showed a decreased level in four patients (P1, P3, P4 and P6) in comparison to controls. The values for the amount of CIV in patients P2 and P5 were near the low borderline values of the controls. The levels of CIV in the patients P1, P2, P3, P4, P5, and P6 were 56%, 76%, 40%, 45%, 86%, and 16% of control values, respectively (Fig. 2B).
Minipig testing
DIA (ab109722, ab109877, Abcam) was used to determine the amounts of CI and CIV in the buccal epithelial cells obtained from a minipig model transgenic for HD. We tested three WT and three TgHD animals The method used provided strong signals in the minpig model cells, and the results can be clearly evaluated.
The inter‑ individual variabilities of the results were obtained, and a uniform trend was not observed within the WT and TgHD groups. The raw data (absolute values) from the analysis showed signal intensity for CI of 78.9 for WT K48, 51.5 for WT K64, and 30.6 for WT K103. The intensity of the signal of the TgHD group was 38.4 for TgHD K63, 25.2 for TgHD K100, and 96.8 for TgHD K104.
The signal intensity of CIV was 111.9 for WT K48, 75.4 for WT K64, and 83.4 for WT K103. In the TgHD group, the signal intensity varied from 58.1 for TgHD K63, crossed to 93.9 for TgHD K100, and then went up to 157.5 for TgHD K104.
Discussion
Although we know the cause of HD and the research in this field is very intensive, neurodegenerative HD is still an incurable disease. One of many important players in HD pathology are the mitochondria and their energy metabolism, which is responsible for the synthesis of more than 90% of ATP for cellular processes. Mitochondrial disturbances in HD are studied primarily in neurons [17], where the impact is most evident and the view is usually functional [18].
The evidence for the involvement of mitochondria in HD comes from post‑mortem, in vivo and in vitro studies. A defect in the activity of the OXPHOS complex IV was found specifically in the caudate and putamen of the brains of HD patients [19], the striatum of mtHtt transgenic (mtHtt‑ Tg) mice [20] or in the muscle of R6/ 2 muscle mice [21]. The mitochondrial‑encoded COX I subunit of CIV was decreased in the platelets of both pre‑HD and symptomatic HD carriers [22]. Complex I was affected in the muscles [23,24] and platelets of HD patients [22,23].
In our study, we focused on mitochondrial disturbances in the peripheral tissues of HD patients, specifically in buccal epithelial cells.We determined the amount of mitochondrial CI and CIV using DIA kits (Abcam). Parallel to patient testing, we also analysed the buccal epithelial cells from a TgHD minipig model. The DIA method that was utilized in our previous study on patients with COX deficiency was optimized for buccal epithelial cells [16].
In our study, in the HD patient samples, we detected a decrease in the amount of CI and/ or CIV in 4/ 6 tested patients in comparison to the controls. The amount of CI varied between 60% and 80% of the control values, and the amount of CIV in four cases was less than 60% of the controls (Fig. 2B). Unfortunately, we do not have any available tests to check the functionality of the oxidative phosphorylation system of buccal cells.However, we can compare the data with similar DIA analysis provided by the fibroblasts of another HD patient group, where the amounts of CI and CIV were lower than 80% of the control values in 6/ 8 patients and the measurement of the specificic activity of CI and CIV did not show any significant differences between patients and controls (data not shown). We suppose that the changes in the CI and CIV protein amounts in the peripheral tissues do not impair function because the enzymes still gain to cover their activities from their reserve capacity. Nevertheless, it could indicate pathological changes connected with HD. The CI and CIV are multi‑subunits, and their synthesis is controlled by both mitochondrial and nuclear DNA. For the proper fully assembled complex holoenzyme, it is necessary for the subunits synthesised in the cytosol to be transported into the mitochondria. It has been shown that mtHtt affects the transport of cytosolic proteins into the mitochondria [25]. In parallel, it is not unreasonable to suggest that the genetic HD mutation may alter nuclear‑ encoded components of the electron transport complexes, resulting in mitochondrial dysfunction. In addition, it is known that there is mtDNA damage in HD [26], and thus, the impaired synthesis of the mitochondrial‑ encoded subunits may contribute to the reduced content of the subunits of the respiratory chain complexes. Silva et al. described a decrease in the mt‑ encoded COX I subunit in the platelets in both pre‑HD and symptomatic HD carriers [22].
The results from the analysis of the amount of CI and CIV in the 36- month‑ old minipig model did not show any differences between WT and TgHD individuals (Fig. 3A, 3B). The probable cause of this is the young age of the tested animals, unlike the HD patients, they are still in the presymptomatic stage of the development of the disease. It will be necessary to carry out this study on a larger group of animals.
The advantage of the method used in this study is that samples of buccal cells can be collected repeatedly without causing stressfor the studied objects. Therefore, this method can serve as a longitudinal monitor of the mitochondria during the asymptomatic stage until the full development of the disease. Especially in TgHD model, buccal smears could be used for monitoring a specific marker of the disease and an eventual development of the therapies.
Although our study has many limitations, the method used is quick, simple and sensitive. It can also be used in other peripheral tissues, such as lymphocytes, muscle or fibroblasts, for the analysis of the amount, and in many cases, the activity of CI and CIV in patients with HD or suspected mitochondrial disease. It can now serve as an additional method for the mapping of mitochondrial disturbances in the peripheral tissues in HD patients.
Our study and its results are not extensive enough for general conclusions, but it could serve as a pilot study for a new direction in the research on the mitochondrial connection to HD.
Acknowledgements
Supported by Czech‑ Norwegian Financial Mecha-nism 2009– 2014 and MSMT under project Contract No 28477/ 2014 – “HUNTINGTON” 7F14308, COST LD15099 (MSMT), the project EXAM from European Regional Program Research and Development Fund – for Innovation Ministry of Education, Youth and Sports ExAM CZ.1.05/ 2.1.00/ 03.0124 (MSMT), GAČR 14- 36804G Centre of Mitochondrial Biology and Pathology (MITOCENTRE) and CHDI Foundation (A‑ 8248), SVV UK 260148/ 2015, PRVOUK‑ P26/ LF1/ 4.
The authors declare they have no potential conflicts of interest concerning drugs, products, or services used in the study.
The Editorial Board declares that the manuscript met the ICMJE “uniform requirements” for biomedical papers.
Accepted for review: 5. 10. 2015
Accepted for print: 23. 10. 2015
RNDr. Hana Hansíková, Ph.D.
Department of Pediatrics and Adolescent Medicine
1st Faculty of Medicine
Charles University and General University Hospital in Prague
Ke Karlovu 2, 128 08 Prague 2
Czech Republic
e-mail: hana.hansikova@lf1.cuni.cz
Sources
1. Purdon SE, Mohr E, Ilivitsky V, Jones BD. Huntington‘s disease: pathogenesis, diagnosis and treatment. J Psychiatry Neurosci 1994; 19(5): 359– 367.
2. The Huntington‘s Disease Collaborative Research Group. A novel gene containing a trinucleotide repeat that is expanded and unstable on Huntington‘s disease chromosomes. Cell 1993; 72(6): 971– 983.
3. Roos RA. Huntington‘s disease: a clinical review. Orphanet J Rare Dis 2010; 5: 40– 40. doi: 10.1186/ 1750‑ 1172‑ 5‑ 40.
4. Brinkman R, Mezei M, Theilmann J, Almgvist E, Hayden MR. The likelihood of being affected with Huntington‘s disease by a particular age, for a specific CAG size. Am J Hum Genet 1997; 60(5): 1202– 1210.
5. Zuccato C, Cattaneo E. Role of brain‑derived neurotrophic factor in Huntington‘s disease. Prog Neurobiol 2007; 81(5– 6): 294– 330.
6. Costa V, Scorrano L. Shaping the role of mitochondria in the pathogenesis of Huntington‘s disease. The EMBO J 2012; 31(8): 1853– 1864. doi: 10.1038/ emboj.2012.65.
7. Damiano M, Diguet E, Malgorn C, D’Aurelio M, Galvan L, Petit F et al. A role of mitochondrial complex II defects in genetic models of Huntington‘s disease expressing N‑terminal fragments of mutant huntingtin. Hum Mol Genet 2013; 22(19): 3869– 3882. doi: 10.1093/ hmg/ ddt242.
8. Milakovic T, Johnson GV. Mitochondrial respiration and ATP production are significantly impaired in striatal cells expressing mutant huntingtin. J Biol Chem 2005; 280(35): 30773– 30782.
9. Waelter S, Boeddrich A, Lurz R, Scherzinger E, Lueder G, Lehrach H et al. Accumulation of mutant huntingtin fragments in aggresome‑like inclusion bodies as a result of insufficient protein degradation. Mol Biol Cell 2001; 12(5): 1393– 1407.
10. Kosinski CM, Schlangen C, Gellerich FN, Gizatullina Z, Deschauer M, Schiefer J et al. Myopathy as a first symptom of Huntington‘s disease in a Marathon runner. Mov Disord 2007; 22(11): 1637– 1640.
11. Mihm MJ, Amann DM, Schanbacher BL, Altschuld RA, Bauer JA, Hoyt KR. Cardiac dysfunction in the R6/ 2 mouse model of Huntington‘s disease. Neurobiol Dis 2007; 25(2): 297– 308.
12. Sassone J, Colciago C, Cislaghi G, Silani V, Ciammola A. Huntington‘s disease: the current state of research with peripheral tissues. Exp Neurol 2009; 219(2): 385– 397. doi: 10.1016/ j.expneurol.2009.05.012.
13. Squitieri F, Falleni A, Cannella M, Orobello S, Ful-ceri F, Lenzi P et al. Abnormal morphology of peripheral cell tissues from patients with Huntington‘s disease. J Neural Transm 2010; 117(1): 77– 83. doi: 10.1007/ s00702‑ 009‑ 0328‑ 4.
14. Van Raamsdonk JM, Murphy Z, Selva DM, Hamidizadeh R, Pearson J, Petersén A et al. Testicular degeneration in Huntington‘s disease. Neurobiol Dis 2007; 26(3): 512– 520.
15. Toneff T, Mende‑ Mueller L, Wu Y, Hwang SR, Bundey R, Thompson LM et al. Comparison of huntingtin proteolytic fragments in human lymphoblast cell lines and human brain. J Neurochem 2002; 82(1): 84– 92.
16. Rodinova M, Trefilova E, Honzik T, Tesarova M, Zeman J, Hansikova H. Non‑ invasive screening of cytochrome c oxidase deficiency in children using a dipstick immunocapture assay. Folia Biol (Praha) 2014; 60(6): 268– 274.
17. Waldvogel HJ, Faull RL. The diversity of GABA(A) receptor subunit distribution in the normal and Huntington‘s disease human brain. Adv Pharmacol 2015; 73: 223– 264. doi: 10.1016/ bs.apha.2014.11.010.
18. Brito V, Giralt A, Enriquez‑Barreto L, Puigdellívol M, Suelves N, Zamora‑ Moratalla A et al. Neurotrophin receptor p75(NTR) mediates Huntington‘s disease‑associated synaptic and memory dysfunction. J Clin Invest 2014; 124(10): 4411– 4428. doi: 10.1172/ JCI74809.
19. Tabrizi SJ, Schapira AH. Secondary abnormalities of mitochondrial DNA associated with neurodegeneration. Biochem Soc Symp 1999; 66: 99– 110.
20. Tabrizi SJ, Workman J, Hart PE, Mangiarini L, Mahal A, Bates G et al. Mitochondrial dysfunction and free radical damage in the Huntington R6/ 2 transgenic mouse. Ann Neurol 2000; 47(1): 80– 86.
21. Gizatullina ZZ, Lindenberg KS, Harjes P, Chen Y, Kosinski CM, Landwehrmeyer BG et al. Low stability of Huntington muscle mitochondria against Ca2+ in R6/ 2 mice. Ann Neurol 2006; 59(2): 407– 411.
22. Silva AC, Almeida S, Laco M, Duarte AI, Dominigues J, Oliveira CR et al. Mitochondrial respiratory chain complex activity and bioenergetic alterations in human platelets derived from pre‑symptomatic and symptomatic Huntington‘s disease carriers. Mitochondrion 2013; 13(6): 801– 809.
23. Parker WD jr, Boyson SJ, Luder AS, Parks JK. Evidence for a defect in NADH: ubiquinone oxidoreductase (complex I) in Huntington‘s disease. Neurology 1990; 40(8): 1231– 1234.
24. Arenas J, Campos Y, Ribacoba R, Martin MA, Rubio JC, Ablando P et al. Complex I defect in muscle from patients with Huntington‘s disease. Ann Neurol 1998; 43(3): 397– 400.
25. Yano H, Baranov SV, Baranova OV, Kim J, Pan Y, Yablonska S et al. Inhibition of mitochondrial protein import by mutant huntingtin. Nat Neurosci 2014; 17(6): 822– 831. doi: 10.1038/ nn.3721.
26. Wang JQ, Chen Q, Wang X, Wang QC, Wang Y, Cheng HP et al. Dysregulation of mitochondrial calcium signaling and superoxide flashes cause mitochondrial genomic DNA damage in Huntington‘s disease. J Biol Chem 2013; 288(5): 3070– 3084. doi: 10.1074/ jbc.M112.407726.
27. Baxa M, Hruska-Plochan M, Juhas S, Vodicka P, Pavlok A, Juhasova J et al. A transgenic minipig model of Huntington‘s Disease. J Huntingtons Dis 2013; 2(1): 47–68.
Labels
Paediatric neurology Neurosurgery NeurologyArticle was published in
Czech and Slovak Neurology and Neurosurgery
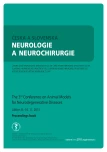
2015 Issue Supplementum 2
- Memantine in Dementia Therapy – Current Findings and Possible Future Applications
- Metamizole vs. Tramadol in Postoperative Analgesia
- Metamizole at a Glance and in Practice – Effective Non-Opioid Analgesic for All Ages
- Memantine Eases Daily Life for Patients and Caregivers
- Advances in the Treatment of Myasthenia Gravis on the Horizon
Most read in this issue
- The Libechov Minipig as a Large Animal Model for Preclinical Research in Huntington’s disease – Thoughts and Perspectives
- Buccal Epithelial Cells as Potential Non‑ invasive Materials for the Monitoring of Mitochondrial Disturbances to Track Huntington‘s Disease Progression – a Pilot Study
- Telemetry Physical Activity Monitoring in Minipig’s Model of Huntington’s Disease
- Grunting in a Genetically Modified Minipig Animal Model for Huntington’s Disease – Pilot Experiments