Effect of the zinc phthalocyanine mediated photodynamic therapy on cytoskeletal apparatus of hela cells
Authors:
Hosikova B 1; Binder S 1; Lenobel R 2; Malohlava J 1; Hosik J 1; Jiravova J 1; Malina L 1; Zapletalova J 1; Kolarova H 1
Authors place of work:
Department of Medical Biophysics, Institute of Molecular and Translational Medicine, Faculty of Medicine and Dentistry, Palacky University, Olomouc, Czech Republic
1; Centre of the Region Hana for Biotechnological and Agricultural Research, Palacky University, Olomouc, Czech Republic
2
Published in the journal:
Lékař a technika - Clinician and Technology No. 2, 2019, 49, 41-45
Category:
Original research
Summary
Photodynamic therapy is a very promising and constantly evolving diagnostic and therapeutic method that is used mainly for malignant and non-malignant tumors treatment. This study deals with the utilization of zinc photosensitizer (λmax ~ 660 nm) from the group of phthalocyanines in photodynamic therapy. The aim of this study is to evaluate in vitro effect of the 5 Jcm-2 zinc phthalocyanine photosensitizer-mediated photodynamic therapy in EC50 concentration (30 nM) on cytoskeletal apparatus of the tumor cell line—HeLa (cervical cancer cells). For the measurement, the tandem mass spectrometry, atomic force and fluorescent confocal microscopy techniques were used. The results showed, that compared to the control cells zinc-derivative mediated photodynamic therapy caused in HeLa cells significant change of the cell height and extensive cytoskeletal actin rearrangement although the levels of beta actin, gamma actin and F-actin did not change significantly. This is probably caused by decreased level of the ARPC2 actin-related protein which is responsible for actin polymerization. Its level decreased 24 hours after therapy by 56%. The cytoskeletal apparatus is one of the basic cellular structures that provides cell shape, cell division and the intracellular transport. After in vitro 5 Jcm-2 zinc derivative-mediated photodynamic therapy, the cervical carcinoma cells showed a significant damage of the cytoskeletal structure followed by changes of cell shape leading to cell death. Considering these results and low effective concentration (EC50 = 30 nM), the therapy used is potentially very promising antitumor treatment.
Keywords:
reactive oxygen species – photodynamic therapy – phthalocyanines – cytoskeletal apparatus
Photodynamic therapy (PDT) is a promising treatment of neoplastic and non-neoplastic diseases. PDT is widely used for treating tumors on the surface of skin or body cavity, including skin tumors, head and areas of the head. This therapeutic approach involves the administration of a tumor-localizing photosensitizer (PS) by intravenous injection or external application, and the subsequent targeted activation of this photo-sensitizer by exposure to light of a specific wavelength. However, PDT is limited by penetration depth of light into human tissues—the longer excitation wavelength the deeper penetration into the target tissue [1, 2]. Depending on the cell type and subcellular localization, PDT has proved to trigger various signaling pathways [3].
PDT is used in technology in clinical trials which make use of artificially built agents and light induced reactive oxygen species (ROS) for medical treatment of malignant cancer. Under normal conditions, ROS are chemically reactive molecules generated during oxygen metabolism in cells. Upon environmental stress, ROS can increase dramatically and can cause severe damage to the cells structures [4]. All amino acid residues of a protein are subject to attack by hydroxyl radicals produced by ionizing radiation; however, Tyr, Phe, Trp, His, Met and Cys residues are the preferred targets. These amino acids are preferentially oxidized when proteins are exposed to ozone. Moreover, under anaerobic conditions, radiolytically generated radicals promote considerable protein- protein cross-linking through •OH-facilitated -S-S- and -Tyr-Tyr- bonding, whereas in the presence of O2, radiolysis leads to considerable fragmentation of the polypeptide chain, probably by the peroxyradical-mediated α amidation pathway [5].
Phthalocyanines are promising second-generation photosensitizers for photodynamic therapy (PDT). Phthalocyanines (Pcs) are a class of tetrapyrrolic macro-cycles related to the naturally occurring porphyrins. Compared with the porphyrin ring, Pcs exhibit an extended 18 π-electron system with four benzene units fused onto the β-pyrrolic positions and nitrogen atoms at the meso-positions instead of the methine bridges in porphyrins. These structural features confer to Pcs a number of properties that make them highly suitable for a variety of applications in biology, medicine and materials science, including strong absorptions and emissions in the near-IR, high stability and easy macrocycle derivatization and high efficiency of generating singlet oxygen [6, 7].
Material and methods
The phthalocyanine zinc photosensitizer (ZnPc) was synthesized by A. Cidlina [8]. This photosensitizer does not aggregate in water due to the presence of eight substituents in non-peripheral positions. Before being used the photosensitizer was diluted in 1X phosphate-buffered saline (PBS). The chemical structure and formula of the phthalocyanine used is presented in the Fig. 1. For all experiments the EC50 concentration was used (EC50 = 30 nM).
Cell lines and culture conditions
Cervical carcinoma cells (HeLa) were tested. Cells were purchased from The European Collection of Authenticated Cell Cultures (ECACC, England). The cells were incubated in Dulbecco´s modified Eagle´s medium (DMEM, Sigma Aldrich, USA) containing the photosensitizer for 24 hours in darkness at 37 °C and 5% CO2.
Light source and exposure
A homemade LED-based light device was used. This source was designed specifically for irradiation of experimental microplates. The samples are illuminated at 660 nm wavelength. The light source is protected by a National Patent CZ 302829 B6. The cells with photosensitizer were exposed to a total irradiation dose 5 Jcm-2 (irradiation time 334 s and intensity of the radiation was 15 mWcm-2) at the room temperature. The control cells represent the non-irradiated cells without photosensitizer.
Atomic force microscopy
For the topography measurement using the atomic force microscope the cells (5·105 per dish) were grown in special glass bottom Petri dishes WillCo (Willco-Wells, Netherlands) in Dulbecco´s modified Eagle´s medium (DMEM) containing the photosensitizing substance for a period of 24 hours in darkness at 37 °C and 5% CO2 atmosphere. The cells were scanned at the room temperature using the Bioscope Catalyst atomic force microscopy (Bruker Daltonics, Germany). The topography image was obtained by PeakForce QNM (Quantitative Nanomechanical Mapping, Bruker Daltonics, Germany) scanning mode at 0.1–0.2 Hz scanning rate and 1000 nm PeakForce amplitude. For imaging, a silicon nitride tip with a resonance frequency of 16–28 kHz and a nominal stiffness constant of 0.12 Nm-1 was used. Images obtained from AFM were processed using Gwyddion 2.40 (Czech Metrology Institute, Czech Republic).
Evaluation of cytoskeletal actin changes using fluorescent microscopy
The cells for fluorescent staining were adhered to the cover glasses. The sterilized cover glass was placed into a Petri dish with the diameter of 35 mm. The cell density for HeLa cell line was 5·104 cells per Petri dish and cell adhesion occurred overnight. After adhesion, the cells were fixed with 4% p-formaldehyde for 1 h at the room temperature. The cell membrane was permeabilized with 0.1% Triton X-100 for 10 min at room temperature. The cytoskeleton actin was fluorescently labeled. For the actin visualization, the Alexa Fluor 532 phalloidin fluorescence probe was used. After permeabilization, the Alexa Fluor 532 Phalloidin probe was applied for 1 h at 37 °C at concentration of 1:200 dissolved in 1xPBS (phosphate buffered saline). After each staining step, the cells were washed twice with 1xPBS. Fluorescence labeling was evaluated using an Axio Observe Z1 confocal microscope (Zeiss, Germany) supplemented with CSU X1 rotating disk (Yokogawa, Japan). An immersion lens of 63 × 1.4 NA was used, excitation of the fluorophores was performed by laser system which offers excitation wavelengths of 405, 488, 532/561 and 639 nm. The fluorescent images were processed in the ImageJ program (Laboratory for Optical and Computational Instrumentation, University of Wisconsin, USA).
Protein analysis
For the protein analysis, the HeLa cells were incubated with photosensitizer at EC50 concentration (30 nM) for the time period of 24 hours and then irradiated. Subsequently, the cells were collected at the appropriate time period—immediately after the irradia-tion, after 4 hours and after 24 hours. The cells were centrifuged for 2 minutes at 1500 rpm. Sub-sequently the supernatant was removed and pellets were stored at –80 °C. The protein concentration was measured through 2-D Quant Kit (InvitrogenTM, USA). The extraction and purification methods were identical with those as described by Petřík [9]. The proteins were labeled followed Boersema et al. [10]. Samples were measured by capillary chromatography and tandem mass spectrometry with ESI ionization (UHR-QTOF maXis, Bruker Daltonics, Germany) on an analytical column filled with RP (reversed phase; C18). Data were collected using the DDA (Data-dependent acquisition) method with cyclic MS (mass spectrometry) collection and variable number of MSMS (tandem MS) specters in the 2 s cycle. Primary data was processed by Data-Analysis (Bruker Daltonics, Germany) and extracted from MGF (files containing a list of precursors and
their fragmentation spectra). Using ProteinScape 3.1, MASCOT (MatrixScience, England) was identified using the HUMAN reference database obtained from
the UNIPROT repository. Protein quantification was performed using a Bruker tool set and the quantitative data was processed using the Perseus 1.3.5 software (Max Planck Institute of Biochemistry, Germany). The relative quantification was calculated as protein level in treated cells sample to protein level in control cells sample.
Statistical analysis
The statistical analysis of changes in topography was performed on 45 control cells and 46 cells treated by 5 Jcm-2 ZnPc PDT using the non-parametrical Mann-Whitney U test.
Results
Atomic force microscopy:
The AFM analysis was performed on HeLa cell treated by 5 Jcm-2 ZnPc PDT using Bruker Bioscope Catalyst atomic force microscopy. The results of topographic changes from the AFM analysis showed that there are significant changes in HeLa cell height after the therapy (see Fig. 2, Table 1).
Table 1: The statistical analysis of the changes in topography using the non-parametrical Mann-Whitney U test. The results are presented as ± SD of 45 control cells and 46 treated cells. A significant difference in height parameter between control cells and 5 Jcm-2 ZnPc PDT treated cells was showed when p = 0.011.
Sample |
Median (µm) |
SD (µm) |
Statistics |
Control
|
3.30 |
0.84
1.10 |
p= 0.011 |
Treated HeLa Cells |
4.31 |
Fluorescent confocal microscopy:
The visualization of the changes in the cytoskeletal actin structure of the on HeLa cells exposed to the
5 Jcm-2 ZnPc PDT was also performed using fluorescent confocal microscopy. The results showed that 5 Jcm-2 ZnPc PDT on HeLa cells led to extensive cytoskeletal actin rearrangement and/or its impairment (see Fig. 3).
Protein analysis:
The results showed that actins beta, gamma and F‑ actin levels did not change significantly at different time periods after the photodynamic therapy. The de-crease of the protein level was evident in the case of ARPC2 protein when 24 hours after therapy its level decreased almost to half in comparison to the immedi-ately collected cells sample (see Fig. 4).
Discussion
In this work, the effect of 5 Jcm-2 ZnPc PDT on the cytoskeletal structure of HeLa cells was studied. The results of topographic changes from the AFM analysis showed that there are significant changes in HeLa cell height after the therapy (p = 0.011). The greater height of the treated cell is probably caused by swelling of the cells after the therapy. These results correspond with the conclusions of Radakovic-Fijan et al. and Agostinist et al. [11, 12]. The difference in organization of the cytoskeletal structure was also observed when visual-izing this structure with Alexa Fluor 532 Phalloidin when reorganization of the microfilament structure is apparent after the therapy. The protein analysis results showed that the levels of actin beta, actin gamma 1 and F-actin proteins did not change significantly at different time intervals. In the case of ARPC2 actin related protein, the level of this protein in the sample decreased 24 hours after the therapy by 56%. Thus, based on the results it can be concluded that the levels of actin proteins have changed minimally, even though the level of ARPC2 protein which is responsible for their polymerization has been reduced by more than half and therefore a change in the cytoskeletal apparatus prob-ably occurred. These conclusions correspond with the conclusions of several authors who also observed the reorganization of the cytoskeletal apparatus after photo-dynamic therapy using several different photosensitive substances [13–17]. Cytoskeletal apparatus is one of the basic cellular structures and provides cell shape, cell division and the intracellular transport. After in vitro 5 Jcm-2 ZnPc PDT, the cervical carcinoma cells showed a significant damage of the cytoskeletal structure leading to cell death.
Conclusion
We conclude that the zinc phthalocyanine-mediated 5 Jcm-2 photodynamic therapy on HeLa cells leads to the disruption of the cytoskeletal apparatus followed by changes of the cell shape leading to cell death which makes this therapy, considering the low effective concentration used (30 nM), potentially promising anti-tumor treatment.
Acknowledgement
The work has been supported by research grants No. LM2015062 Czech-BioImaging, IGA_LF_2019_004 and LO1304. The authors would like to thank the Department of Pharmaceutical Chemistry and Drug Control, Faculty of Pharmacy, Charles University in Prague, Hradec Králové, Czech Republic for providing the photosensitizers used in this study.
Barbora Hošíková, MSc
Department of Medical Biophysics
Faculty of Medicine and Dentistry
Palacky University in Olomouc
Křížkovského 511/8, 771 47 Olomouc
E-mail: barbora.hosikova@upol.cz
Phone: +420 585 632 200
Zdroje
- Yin H, Ye X, Li Y, Niu Q, Wang C, Ma W. Evaluation of the effects of systemic photodynamic therapy in a rat model of acute myeloid leukemia. Journal of Photochemistry and Photobiology B: Biology. 2015 Dec;153:13-9.
- Mustafa FH, Jaafar MS. Comparison of wavelength-dependent penetration depths of lasers in different types of skin in photo-dynamic therapy. Indian Journal of Physics. 2013 Mar;87:203-9.
- Tsaytler PA, C O'Flaherty M, Sakharov DV, Krijgsveld J, Egmond MR. Immediate protein targets of photodynamic treatment in carcinoma cells. Journal of proteome research. 2008 Sep;7(9):3868-78.
- Qi D, Wang Q, Li H, Zhang T, Lan R, Kwong DW, et al. SILAC-based quantitative proteomics identified lysosome as a fast response target to PDT agent Gd-N induced oxidative stress in human ovarian cancer IGROV1 cells. Molecular BioSystems. 2015 Nov;11(11):3059-67.
- Stadtman ER. Protein oxidation and aging. Free radical research. 2006 Dec;40(12):1250-8.
- Liu JY, Li J, Yuan X, Wang WM, Xue JP. In vitro photodynamic activities of zinc(II) phthalocyanines substituted with pyridine moieties. Photodiagnosis and photodynamic therapy. 2016 Mar;13:341-3.
- Ongarora BG, Zhou Z, Okoth EA, Kolesnichenko I, Smith KM, Vicente MG. Synthesis, spectroscopic, and cellular properties of α-pegylated cis-A2B2- and A3B-types ZnPcs. Journal of porphyrins and phthalocyanines. 2014 Oct-Nov;18(10-11):1021-33.
- Cidlina A. Syntéza kationických ftalocyaninů. [Diploma thesis]: Charles University in Prague, Faculty of Pharmacy in Hradec Kralove. 2011. 72p.
- Petřík I. Analýza proteinových fosforylací proteomickými metodami. [Bachelor thesis]: Palacky University in Olomouc, Faculty of Science. 2013. 62p.
- Boersema PJ, Raijmakers R, Lemeer S, Mohammed S, Heck AJ. Multiplex peptide stable isotope dimethyl labeling for quanti-tative proteomics. Nature protocols. 2009 Mar;4(4):484-94.
- Radakovic-Fijan S, Rappersberger K, Tanew A, Hönigsmann H, Ortel B. Ultrastructural changes in PAM cells after photo-dynamic treatment with delta-aminolevulinic acid-induced porphyrins or photosan. The Journal of investigative dermatology. 1999 Mar;112(3):264-70.
- Agostinis P, Berg K, Cengel KA, Foster TH, Girotti AW, Gollnick SO, et al. Photodynamic therapy of cancer: an update. CA: a cancer journal for clinicians. 2011 Jul-Aug;61(4):250-81.
- Welch MD, Iwamatsu A, Mitchison TJ. Actin polymerization is induced by Arp2/3 protein complex at the surface of Listeria monocytogenes. Nature. 1997 Jan;385(6613):265-9.
- Pacheco-Soares C, Maftou-Costa M, DA Cunha Menezes Costa CG, DE Siqueira Silva AC, Moraes KC. Evaluation of photo-dynamic therapy in adhesion protein expression. Oncology letters.2014 Aug;8(2):714-8.
- Ferreira SD, Tedesco AC, Sousa G, Zângaro RA, Silva NS, Pacheco MT, et al. Analysis of mitochondria, endoplasmic reticulum and actin filaments after PDT with AlPcS(4). Lasers
in medical science. 2004 Jan;18(4):207-12. - Tsai T, Ji HT, Chiang PC, Chou RH, Chang WS, Chen CT. ALA-PDT results in phenotypic changes and decreased cellular invasion in surviving cancer cells. Lasers in surgery and medicine. 2009 Apr;41(4):305-15.
- Juarranz A, Villanueva A, Díaz V, Cañete M. Photodynamic effects of the cationic porphyrin, mesotetra(4N-methyl-pyridyl)porphine, on microtubules of HeLa cells. Journal of photochemistry and photobiology. B, Biology. 1995;27(1):47-
Štítky
BiomedicineČlánok vyšiel v časopise
The Clinician and Technology Journal
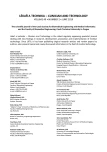
2019 Číslo 2
Najčítanejšie v tomto čísle
- Effect of the zinc phthalocyanine mediated photodynamic therapy on cytoskeletal apparatus of hela cells
- Methods evaluating upper arm and forearm movement during a quiet stance
- Wrist rehabilitation with manipulator to perform passive and active exercises
- A framework to assess mechanics of stump–socket interaction in transfemoral amputees