Introducing in vivo pancreatic cancer models for the study of new therapeutic regimens
Zavedení in vivo modelů karcinomu pankreatu pro studium nových terapeutických režimů
Úvod: Karcinom pankreatu je závažné onkologické onemocnění se stále se zvyšující incidencí a vysokou mírou morbidity a mortality. Terapeutické možnosti jsou limitované a pětileté přežití je 7–20 %, v závislosti na možnosti chirurgické resekce a časnosti záchytu onemocnění. Většina nemocných s touto diagnózou umírá v důsledku rezistence nádorových buněk a mikroprostředí vůči používané léčbě.
Metody: V naší práci jsme se zaměřili na zavedení dvou typů in vivo modelů, kterými jsou cell-line derived xenograft (CDX) a patient derived xenograft (PDX). Tyto dva modely se navzájem výrazně liší metodologicky, náročností technickou i finanční, ale i dosaženými výsledky.
Výsledky: V pilotní studii se nám podařilo úspěšně zavést CDX model s velmi agresivní a rezistentní linií PaCa-44 karcinomu pankreatu o celkovém počtu 30 jedinců NU/NU myší. Dále jsme vytvořili tři PDX modely s různými subtypy karcinomu pankreatu, pacientů operovaných na Chirurgické klinice FNKV, s jejich následnou retransplantací do dalších generací o celkovém počtu 23 jedinců kmene NOD/SCID a 47 jedinců kmene NU/NU. Zavedené CDX i PDX modely pak slouží k porovnání jak konvenčních, tak experimentálních chemoterapeutických režimů.
Závěr: Dalšími kroky bude vyhodnocení vlivu léčebných režimů pomocí zobrazovacích i molekulárně genetických metod a optimalizace celého procesu pro využití v rámci precizní personalizované léčby pacientů s karcinomem pankreatu. Nadcházejícím cílem je vytvořit knihovnu PDX modelů nejčastějšího duktálního adenokarcinomu pankreatu a dalších vzácných subtypů karcinomu pankreatu.
Klíčová slova:
zvířecí modely – experimentální chirurgie – karcinom pankreatu – experimentální terapie – patient derived xenograft
Authors:
Sychra T 1,2; Václavíková R 3,5; Szabó A 2,4; Spálenková A 2,3,5; Šeborová K 3,5; Balatka Š 3,5; Tesařová T 3,5; Kočí K 2; Gürlich R 1,2; Souček P 3,5; Oliverius M 1,2
Authors place of work:
Chirurgická klinika 3. lékařské fakulty Univerzity Karlovy a Fakultní nemocnice Královské Vinohrady, Praha
1; 3. lékařská fakulty Univerzity Karlovy
2; Centrum toxikologie a zdravotní bezpečnosti, Státní zdravotní ústav, Praha
3; Ústav patologie 3. lékařské fakulty Univerzity Karlovy a Fakultní nemocnice Královské Vinohrady, Praha
4; Biomedicínské centrum, Lékařská fakulta Univerzity Karlovy v Plzni
5
Published in the journal:
Rozhl. Chir., 2022, roč. 101, č. 12, s. 584-592.
Category:
Původní práce
doi:
https://doi.org/10.33699/PIS.2022.101.12.584–592
Summary
Introduction: Pancreatic cancer is a severe oncological disease with an ever-increasing incidence and a high rate of morbidity and mortality. Therapeutic options are limited and the five-year overall survival rate is 7–20%, depending on the possibility of surgical resection and the earliness of detection. Most patients with this diagnosis die due to the resistance of tumour cells and their microenvironment to the used treatment regimes.
Methods: In our study, we focused on the implementation of two in vivo models, which are the cell-line derived xenograft (CDX) and the patient-derived xenograft (PDX). These two models differ significantly from each other methodologically, technically, financially, but also in their achieved results.
Results: In a pilot study, we managed to successfully implement the CDX model with a very aggressive and resistant PaCa-44 line of pancreatic cancer in a total of 30 NU/NU strain mice. Furthermore, we created three PDX models with various subtypes of pancreatic cancer from patients operated at the University Hospital Kralovske Vinohrady, Department of General Surgery. These tumours were re-transplanted into subsequent generations of 23 individuals of NOD/SCID strain and 47 NU/NU strain mice. The established CDX and PDX models are then used to compare conventional and experimental chemotherapy regimens.
Conclusion: The next steps will be to evaluate the effects of treatment regimens by using imaging and molecular genetic methods and to optimise the entire process for further use in precise personalised medicine for patients with pancreatic cancer. The upcoming goal is to create a library of PDX models of the most common pancreatic ductal adenocarcinoma and other rare subtypes of pancreatic cancer.
Keywords:
experimental surgery – Animal models – Pancreatic cancer – experimental therapies – patient derived xenograft
INTRODUCTION
Pancreatic cancer is a severe oncological disease with an ever-increasing incidence and a high rate of morbidity and mortality. Between 1990 and 2017, the number of deaths and the incidence of pancreatic cancer more than doubled worldwide [1]. This trend is very likely to continue due to the ageing of the population, the increasing incidence of this disease at a young age, and the rising standard of living [2].
Therapeutic options are limited, and the five-year survival in various studies is around 7–20%, depending on the possibility of surgical resection and an early detection of the disease. However, most patients die as a result of this diagnosis [3,4]. This is mainly due to the resistance of tumour cells and their low sensitivity to the treatment used in patients with other solid tumours.
The tumour microenvironment (TME) of pancreatic cancers, consisting of immune cells, fibroblasts, cytokines, metabolites and many other components, is very likely responsible for the failure of single-component cytotoxic therapy. A slight improvement in overall survival (OS) was achieved with the use of multicomponent cytotoxic therapy between 2000 (5.26%) and 2020 (10%). Based on a recently published study by Park et al. the indication of adjuvant radiotherapy remains controversial due to its unconvincing effect on prolonging OS [5].
The only curable therapeutic option is surgical resection, indicated only as a primary treatment in the early stages which are detected only in a small number of cases. The latest clinical guidelines (National Comprehensive Cancer Network; European Society for Medical Oncology) recommend neoadjuvant chemotherapy for all patients who do not have a tumour smaller than 2 cm and with a negative lymph node finding, in order to achieve radical operability. According to the local finding of the tumour and the overall state of the patient (PST – performance status), the m/FOLFIRINOX regimen (Oxaliplatin, Irinotecan, Leucovorin, 5-Fluorouracil) is then chosen preferentially, however, in case of worse PST, this regimen has currently been replaced by gemcitabine with nab-paclitaxel or capecitabine. Gemcitabine alone is used as a last resort. In most cases, patients are subsequently recommended for adjuvant chemotherapy [6,7].
The aim of this study was to provide a detailed description of the methodology for the implementation of two types of in vivo models, cell-line derived xenografts (CDX) and patient-derived xenografts (PDX), at the Experimental Animal Welfare Laboratory of the National Institute of Public Health (NIPH) in Prague. PDX models were established in cooperation with the Department of General Surgery, Third Faculty of Medicine, Charles University (3FM CU) in Prague, University Hospital Kralovske Vinohrady (UHKV) in Prague and the Department of Pathology, 3FM CU and UHKV in Prague for subsequent use in preclinical research of experimental chemotherapeutics and personalised medicine. These two models differ significantly from one another in terms of methodology, technical and financial complexity, but also in achievable results (Tab. 1). The difference is caused mainly by the absence of natural TME in CDX models which are based on the application of monoculture cells of standardised tumour lines in the in vivo model. On the other hand, the implementation of PDX models is based on the implantation of a part of the patient’s tumour – that is a heterogeneous population of tumour cells, fibroblasts, immune cells, endothelium, stroma, etc., which creates a real tumour environment corresponding to the given patient.
METHODS
All experimental work with animals was carried out, implemented in compliance with and governed by the existing regulations and guidelines for the breeding and experimental use of animals in accordance with Act No. 246/1992 Coll., on the protection of animals against cruelty, as amended, and performed by persons with authorization to work with experimental animals. Immunodeficient strains of mice were used for the experiments at the Experimental Animal Welfare Department of the NIPH in a specific pathogen-free environment. Procedures of approved experimental projects with CDX (Study of genetic factors affecting the biological behaviour and therapy of tumour models on xenografts; MZDR 20330/2018-4/OVZ) and at PDX (Experimental model of an immunodeficient mouse with human pancreatic cancer, MZDR 37099 /2021-5/OVZ) models were followed. Animals were provided with immunodeficient mouse feeding and fluids ad libitum. The study was approved by the Ethics Committee of the UHKV.
Anaesthesia and marking of individuals
After being placed in the Animal Welfare Department of the NIPH, the experimental animals had a two-week acclimatisation period, during which the individuals were not handled or otherwise intervened with the exception of changing the bedding and replenishing food and fluids. After this period, all interventions were performed under general inhalation isoflurane anaesthesia (GA), with the exception of intravenous (i.v.) application. Operative procedures exceeding the level of pain of a needle injection were treated afterwards by the subcutaneous (s.c.) application of the analgesic Meloxicam (5 MG/ ML INJECTION SOLUTION; Bioveta, a.s.; Ivanovice na Hane, Czech Republic) at a dose of 2 mg/kg. The RWD anaesthetic kit consists of an oxygenator, a flow meter, a vaporizer, inlet and outlet tubes, an induction chamber, an inhalation mask, two isoflurane filters, a device for exhausting excess anaesthetic and a heated pad. Introduction to anaesthesia was performed at a flow rate of 3 l/min with a 3% fraction of isoflurane (ISOFLURIN 1000 mg/g; VETPHARMA ANIMAL HEALTH, S.L.; Barcelona; Spain); interventions were performed on an inhalation mask with the same flow rate and 1.5–2.5% isoflurane fraction. Immediately after placing the individual on the mask, eye ointment (OPHTHALMO-AZULEN 7.5mg/g; Zentiva, k. s.; Prague; Czech Republic) was applied to prevent damage to the animal’s eyes.
Marking of individuals was done by tattooing the ears under GA with a tattoo needle (Liner 5; 0.30 mm LT) and different colours (Mom´s; Millenium Colors, Inc; USA) (Fig. 1). Each individual was marked with a unique combination of colour and number of dots so that individuals could not be confused within one group or between individual experimental groups.
In vivo tumour models
Cell-line derived xenografts (CDX)
The methodology of experiments with CDX in vivo models was established in the NIPH Toxicogenomic laboratories and is in detail described in previously published studies [8,9]. To create these models, a suspension of cells of standardised lines of pancreatic cancer (specifically PaCa-44 with KRAS12V and TP53176S mutations) was applied subcutaneously onto one side of the back area with 1–5×106 cells in 100 μl of vehicle (Fig. 2) in immunodeficient NU/NU mice (Athymic Nude mice, Crl:NU(NCr)-Foxn1nu). It takes approximately 14 days for the tumour to grow to the required size of at least 100 mm3.
Patient-derived xenografts (PDX)
Immunodeficient NOD/SCID mice (JAXTM Mice strain: NOD SCID; NOD.CB17-Prkdcscid/J) were used in the introduction of PDX models. One day before the actual implantation, the removal of fur from the back of the mice of the F0 generation was done. Firstly, the top layer of fur was removed with a clipper and then the removal of the undercoat was completed by chemical depilation using a depilatory cream (Balea; Karlsruhe, Germany).
Successful attachment of the tumour depends on the perfect time management of a team composed of different expertise and work roles (surgeon, pathologist, animal laboratory personnel) on the day of implantation. All enrolled patients from whom we obtained tumour tissue first signed an informed consent. A sample of tumour tissue was obtained from a hemipancreatoduodenetectomy, distal or total pancreatectomy.
Immediately after removing the resected tissue from the patient’s body, it was taken to the pathology laboratory without fixation fluid. The tumour tissue was macroscopically identified using information of the predicted location based on imaging data, visual and palpation examination. A part of the tumour tissue with a volume of approx. 5–10 mm3 was safely removed by the pathologist, then immediately placed into culture medium (RPMI 1640 medium with L-Glutamine (300 mg/l), NaHCO3 (2.0 g/l), penicillin (1000 U/ ml), streptomycin (100 μg/ml), sodium pyruvate (1mM) and HEPES buffer (15mM)) tempered at 2–8°C so that the definitive histopathological conclusion could not be affected in any way. Furthermore, samples of tumour tissue and non-tumour-affected (control) pancreatic tissue were also taken for further processing (e.g. determination of genetic profiles using sequencing methods) and immediately frozen at -80°C.
The tissue sample for implantation was then transported from the pathology laboratory to the animal laboratory, where everything had been already prepared for implantation (see Tab. 2). Under strictly aseptic conditions bilateral incisions of approx. 1–3 mm in the back area were made to the individuals of the F0 generation, which were pre-treated with an antiseptic preparation (povidone iodine; (Braunol; B.Braun Melsungen, Germany). A pocket was bluntly dissected in the subcutaneous tissue with a size of approx. 1 cm2 (Fig. 3), where the tumour tissue with a volume of approx. 1–3 mm3 was inserted (Fig. 4). The incisions were closed with individual sutures using a non-absorbable monofilament fibber (Covidien SURGIPRO II Monofilament Polypropylene 6-0; Dublin, Ireland) and a liquid dressing (2% iodine- collodion) was applied to the wounds (Fig. 5). Then the analgesic Meloxicam (5 MG/ML INJECTION SOLUTION; Bioveta, a.s.; Ivanovice na Hane, Czech Republic) was applied subcutaneously in a dose of 2 mg/kg. After the procedure, the animal was placed in a separate ubication for observation and evaluation of the clinical condition, possible signs of failure to thrive or pain.
In addition, basic biological parameters were monitored such as the behaviour of the individuals, their regular weighing was done and photo documentation was taken, and any change in the size of the tumour growth and in relation to the administered protocol treatment was also monitored. We perform the measurement using a digital calliper, measuring the shortest and longest dimension of the tumour. In the active treatment generation, we have used 3D imaging of the tumour topography with a Peira TM900 measuring scanner (Peira, Turnhout, Belgium).
Once the tumour has grown to the required volume of at least 100 mm3, the individual of the F0 generation was killed using carbon dioxide while still under the influence of GA, followed by an autopsy. The tumour was divided and tumour tissue with a volume of approximately 1–3 mm3 was implanted in the back area of 3–5 individuals of the F1 generation in the same way as described above. At each re-transplantation, a portion of the tumour tissue sized of 20–50 mm3 was inserted into a fixation medium (10% formalin) for histopathological verification of the nature and structure of the tumour. Another part was taken and frozen at -80°C for further molecular analyses.
After tumour growth in the F1 generation, re-transplantation into the therapeutic F2 generation of 20–40 individuals was performed in the same way as described above, thus a statistically valid number of individuals was reached, exactly according to the diagram (Fig. 10). Afterwards it was possible to administer conventional and experimental chemotherapy regimens to the F2 generation and determine their effect and toxicity based on the clinical status of the individuals, the size of the tumour, and any molecular changes in the blood, tumour and the tissue of other organs. Chemotherapeutic agents can be administered intraperitoneally (i.p.) (Fig. 7) or intravenously (Fig. 8).
RESULTS
In our study, we managed to implement a CDX model with the PaCa-44 line of pancreatic cancer with a total number of 30 individuals of NU/NU mice as well as a PDX model created directly from a tumour fragment of a patient with pancreatic cancer. This PDX model was successfully re-transplanted from the F0 generation to subsequent generations with a total number of 20 individuals of the NOD/SCID strain and 47 individuals of the NU/NU strain. In addition, other types of pancreatic cancer have been successfully implanted from patients operated on at the Department of General Surgery, 3FM CU and UHKV, which shows that the procedure was reliably established.
In the CDX trial with the standardised PaCa-44 pancreatic cancer line, the attachment success rate was 100%, the tumour attached and grew in all 30 individuals. PDX models, on the other hand, usually have a much lower success rate, which depends on the type of implanted cancer and the logistics of the team. In our case, the total success rate of all types of pancreatic carcinoma in NOD/SCID strains was 95.83% (23 out of 24 individuals in generations F0 to F2) and NU/NU strain 85.45% (47 out of 55 individuals in generations F2 to F4). In the first PDX trial of pancreatic cancer, it took an average of 40 days for the tumour to grow to 100–150 mm3.
The time interval from the removal of the resected tissue from the patient’s body to the implantation of the tumour is crucial for the successful attachment of the tumour fragment and the formation of the tumour in the mouse model. In our case, this interval was 54 minutes at the beginning of our work with PDX in vivo models compared to the current 36 minutes. The intervals from removing the resected tumour tissue from the patient’s body to placing the sample in the tempered culture medium ranged from 10 to 19 minutes.
Currently, our research team disposes with PDX models of three different types of pancreatic cancer (ductal adenocarcinoma, adenosquamous and acinar) that can be used for therapeutic intervention, monitoring the effectiveness of experimental chemotherapeutics compared to conventional cytostatics, and identifying molecular biomarkers and therapeutic targets for the development of new treatments.
DISCUSSION
The history of the creation of the first CDX and PDX models is connected with the discovery of immunodeficient mice in 1960, specifically NU/NU mice, which do not have a thymus and thus have an associated T-cell immunity disorder. This strain of mice is still widely used in research due to its relatively low price, tameness, the high success rate of tumour attachment and bare skin, which is advantageous for monitoring the growth of subcutaneous tumours (Fig. 2). After 1990, more immunocompromised strains were created, including NOD/ SCID (non-obese diabetic/severe combined immune deficiency) mice with impaired function of T, B and NK immune cells. (Fig. 1) [10]
In the collected samples, molecular genetic changes can be monitored in connection with changes in the tumour mass, the effects and manifestations of the toxicity of the administered treatment, especially changes in the gene and protein expression of key cell signalling pathways with a special focus on the RAS signalling pathway and possibly on crucial genes in the development of resistance and effectiveness of administered drugs. The molecular stability of the tumour is also monitored by comparing the original sample from the patient with generations before and after treatment. From the point of view of the genetic profile, which can be studied in detail with today’s approaches, in 95% of pancreatic cancers, mutated KRAS oncogene could be found, which is also assumed to be the main initiating and controlling mechanism of tumour cell proliferation and survival [3]. For this reason, inhibition of the RAS signalling pathway appeared as a promising treatment of ductal adenocarcinoma, but all results published so far were unsuccessful or ultimately insignificant [11]. Finding the most suitable effective drugs and modified therapeutic regimens, as well as new cellular therapeutic targets, that would also be effective in cases of resistant tumours, is currently a possibility potentially leading to improved treatment outcomes of this severe malignant disease [12].
The development of new drugs goes through a complex and very expensive process in terms of time and money, including the costs of basic research, the conduction of subsequent clinical trials and the introduction of a new drug to the market. Simply, newly discovered and synthesised substances in basic research enter the preclinical part of the research, in which their pharmacological screening and monitoring of toxicity are carried out both at the cellular level using in vitro methods and at the level of the whole organism in so-called in vivo models. Experiments on the whole organism using in vivo models are necessary to verify the effect of the drug on organ systems with all the links, side effects and manifestations of acute and chronic systemic toxicity (FDA development process, [10]). Selected effective preparations based on these procedures are then suggested for further testing. Therefore, for the purposes of testing the effects and systemic toxicity of experimental chemotherapeutic drugs, the study of their effectiveness and systemic toxicity in the above mentioned in vivo models is essential.
Our team has succeeded in establishing a methodology for the preparation and reproduction of CDX and PDX in vivo models with a very high success rate. In the available literature, the success rate of PDX attachment varies in a very wide range (20–80%) depending on the histological type and aggressiveness of the tumour, however, the dispersion of success differs even for histologically identical carcinomas between individual research groups. Specifically, in ductal adenocarcinoma of the pancreas, in various studies, the success rate of attachment is around 60–80% [13,14]. The rate of growth of the tumour to the required size also depends on the type of pancreatic cancer implanted, however, the rate of growth accelerates in individual generations [10,13].
Procedures for the administration of both conventional and experimental chemotherapy have been established and tested, which is essential for comparability with patient conditions and for limiting the systemic toxicity of the regimens. Last but not least, processes were created to verify the effectiveness of therapeutic interventions. At the same time, there is a consensus in the literature about preserving the heterogeneity of the tumour microenvironment and the genetic and histological character of the patient’s tumour in individual generations of PDX models. For this reason, there is an undeniable potential and importance for the use of PDX models in clinical trials of new pharmaceuticals (increase in predictability by 80%), the search for diagnostic, therapeutic and prognostic biomarkers in precise personalised medicine, in contrast to the current “one size fits all” approach to chemotherapy treatment [10,15]. These considerations are evidenced, for example, by the use of these models to understand the individual sensitivity of patients to adjuvant gemcitabine chemotherapy at the molecular, immunohistochemical and macroscopic levels [16]. PDX models have already been able to provide evidence of clinical variation in sensitivity to cisplatin and gemcitabine chemotherapy in pancreatic cancers with BRCA1 and BRCA2 mutations [17].
A certain negative is the financial, time and ethical demands of PDX models. Buying, breeding and caring for immunodeficient mice is expensive and relatively sophisticated work with living creatures, requiring special premises and an experienced team. The very success of implantation depends on the cooperation of several experts in a short time sequence and on good preparation of the experiment. For the successful use of PDX models for the needs of personalised medicine, it is also necessary to quickly create a sufficient number of individuals before the tumour itself causes the death of the patient, which is probably the main limitation in the case of pancreatic cancer. Immunity, which is compromised to varying degrees in immunodeficient mice, plays a crucial role in the fight against cancer, thus the scientists have to always take this fact in consideration when evaluating the results.
For the reasons described above, histopathological, molecular genetic and other analyses will be performed with the aim of verifying and comparing the character of the tumour tissue of the original tumour and with individual generations of in vivo models in our conditions. At the same time, the effectiveness of conventional and experimental chemotherapy regimens will be verified, compared and determined in therapeutic generations with the aim of obtaining objective data on the most suitable treatment regimen for the next phases of clinical research.
Other goals will be the identification of significant molecular changes that accompany the application of these regimens and the assessment of their use as molecular biomarkers for evaluating the effectiveness of treatment and possibly as potential therapeutic targets for achieving higher efficiency of therapy for patients with pancreatic cancer.
CONCLUSION
The use of in vivo models in oncological research for testing of new substances with an antitumour effect is very current. Our team succeeded in implementing and starting to use two in vivo models (CDX and PDX) with different subtypes of primary pancreatic cancers. We described our methodology in detail in this article. The upcoming goal is to create a library of PDX models of the most common type of pancreatic carcinoma and other rare subtypes of pancreatic cancer obtained from patients of the Department of General Surgery, 3FM CU and UHKV. This will allow us, for the needs of personalised therapy, to test and subsequently recommend individualised therapeutic regimens for a specific tumour subtype. At the same time, we aim to investigate regimens containing new experimental chemotherapeutics, possibly targeted drugs, and to search for diagnostic, therapeutic, prognostic and other biomarkers for precision oncology.
Abbreviation:
GA – general anaesthesia
CDX – cell-lines derived xenograft
OS – overall survival
PDX – patient derived xenograft
PST – performance status
s.c. – subcutaneous
i.v. – intravenous
i.p. – intraperitoneal
TME – tumour microenvironment
NIPH – National Institute of Public Health
3FM – 3rd Faculty of Medicine
CU – Charles University
UHKV – University Hospital Královské Vinohrady
Acknowledgement
A big acknowledgement goes to the team of nurses and paramedics of the Department of General Surgery, 3FM CU and UHKV, led by the station nurse of the operating rooms, Mgr. Martina Rumlova, whose help with management is invaluable. Many thanks also go to the staff of the Department of Pathology of 3FM CU and UHKV for their analysis and characterization of tumour tissue samples and to all other staff involved in the development of in vivo models of pancreatic cancer.
Funding:
Donatio Facultatis Medicae Tertiae, MŠMT project of the programme INTER-EXCELLENCE, subprogramme INTER-ACTION No. LTAUSA19032, GAČR project No. 21- 14082S and AZV project No. NV19-08-00113.
Conflict of interests
The authors declare that they have not conflict of interest in connection with this paper and that the article has not been published in any other journal, except congress abstracts and clinical guidelines.
MUDr. Tomáš Sychra
Department of General Surgery
3rd Faculty of Medicine, Charles University
University Hospital Kralovské Vinohrady
e-mail: tomas.sychra@lf3.cuni.cz
ORCID: 0000-0002-6239-5779
Rozhl Chir. 2022;101:584–592
Zdroje
1. The global, regional, and national burden of pancreatic cancer and its attributable risk factors in 195 countries and territories, 1990–2017: a systematic analysis for the Global Burden of Disease Study 2017. Lancet Gastroenterol Hepatol. 2019 Dec;4(12):934–947. doi: 10.1016/S2468- 1253(19)30347-4.
2. Rahib L, Smith BD, Aizenberg R, et al. Projecting cancer incidence and deaths to 2030: The unexpected burden of thyroid, liver, and pancreas cancers in the United States. Cancer Res. 2014 Jun 1;74(11):2913–2921. doi: 10.1158/0008- 5472.CAN-14-0155.
3. Bannoura SF, Uddin H, Nagasaka M, et al. Targeting KRAS in pancreatic cancer: new drugs on the horizon. Cancer Metastasis Rev. 2021 Sep;40(3):819–835. doi: 10.1007/s10555-021-09990-2.
4. Macedo FI, Ryon E, Maithel SK, et al. Survival outcomes associated with clinical and pathological response following neoadjuvant FOLFIRINOX or gemcitabine/ nab-paclitaxel chemotherapy in resected pancreatic cancer. Ann Surg. 2019 Sep;270(3):400–413. doi: 10.1097/ SLA.0000000000003468.
5. Park W, Chawla A, O’Reilly EM. Pancreatic cancer: A review. JAMA 2021;326(9):851– 862. doi: 10.1001/jama.2021.13027.
6. Gemenetzis G, Groot VP, Blair AB, et al. Survival in locally advanced pancreatic cancer after neoadjuvant therapy and surgical resection. Ann Surg. 2019 Aug;270(2):340–347. doi: 10.1097/ SLA.0000000000002753.
7. Janssen QP, van Dam JL, Doppenberg D, et al. FOLFIRINOX as initial treatment for localized pancreatic adenocarcinoma: A retrospective analysis by the Trans-Atlantic Pancreatic Surgery Consortium. JNCI 2022 May;114(5):695–703. doi: 10.1093/jnci/djac018.
8. Mohelnikova-Duchonova B, Kocik M, Duchonova B, et al. Hedgehog pathway overexpression in pancreatic cancer is abrogated by new-generation taxoid SB-T-1216. Pharmacogenomics Journal 2017 Oct;17(5):452–460. doi: 10.1038/ tpj.2016.55.
9. Oliverius M, Flasarova D, Mohelnikova- Duchonova B, et al. KRAS pathway expression changes in pancreatic cancer models by conventional and experimental taxanes. Mutagenesis 2019 Sept;34(5–6):403–411. doi: 10.1093/mutage/ gez021.
10. Goto T. Patient-derived tumor xenograft models: Toward the establishment of precision cancer medicine. Journal of Personalized Medicine 2020 Sep;10(3):1–14. doi: 10.3390/jpm10030064.
11. Uprety D, Adjei AA. KRAS: From undruggable to a druggable cancer target. Cancer Treat Rev. 2020 Sep;89:102070. doi: 10.1016/j.ctrv.2020.102070.
12. Nicolaou KC. Advancing the drug discovery and development process. Angewandte Chemie – International Edition 2014;53(35):9128–9140. doi: 10.1002/ anie.201404761.
13. Wang Y, Cui J, Wang L. Patient-derived xenografts: A valuable platform for clinical and preclinical research in pancreatic cancer. Chinese Clinical Oncology 2019;8(2). doi: 10.21037/cco.2019.02.04.
14. Kim MP, Evans DB, Wang H, et al. Generation of orthotopic and heterotopic human pancreatic cancer xenografts in immunodeficient mice. Nature Protocols 2009;4(11):1670–1680. doi: 10.1038/ nprot.2009.171.
15. Garcia PL, Miller AL, Yoon KJ. Patient-derived xenograft models of pancreatic cancer: Overview and comparison with other types of models. Cancers 2020;12(5):1327. doi: 10.3390/cancers12051327.
16. Xue A, Julovi SM, Hugh TJ, et al. A patient- derived subrenal capsule xenograft model can predict response to adjuvant therapy for cancers in the head of the pancreas. Pancreatology 2015;15(4):397–404. doi: 10.1016/j.pan. 2015.04.008.
17. Lohse I, Borgida A, Cao P, et al. BRCA1 and BRCA2 mutations sensitize to chemotherapy in patient-derived pancreatic cancer xenografts. British Journal of Cancer 2015;113(3):425–432. doi: 10.1038/ bjc.2015.220.
Štítky
Chirurgia všeobecná Ortopédia Urgentná medicínaČlánok vyšiel v časopise
Rozhledy v chirurgii
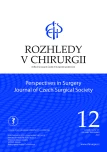
2022 Číslo 12
- Metamizol jako analgetikum první volby: kdy, pro koho, jak a proč?
- Kombinace metamizol/paracetamol v léčbě pooperační bolesti u zákroků v rámci jednodenní chirurgie
- Fixní kombinace paracetamol/kodein nabízí synergické analgetické účinky
Najčítanejšie v tomto čísle
- Tepny hlavy a krku prasete domácího z pohledu zobrazovacích metod a experimentální chirurgie
- Léčba chylothoraxu embolizací hrudního mízovodu
- Introducing in vivo pancreatic cancer models for the study of new therapeutic regimens
- Permanentní žilní přístup v experimentální chirurgii – naše zkušenosti