Hsp90 – a Target for Anticancer Therapy
Authors:
E. Růčková; P. Müller; B. Vojtěšek
Authors‘ workplace:
Masarykův onkologický ústav, Brno
Published in:
Klin Onkol 2011; 24(5): 329-337
Category:
Reviews
Overview
Molecular chaperones help other proteins to achieve and maintain their proper conformation. Chaperones bind to newly synthesized or unfolded polypeptide chains, actively modify their conformation and participate on their transport or degradation. Chaperones play an important role in cancer cell, where their increased activity enables stabilization of many mutant proteins and overcoming the stress generated by genetic instability. Hsp90 represents a key chaperone in cancer cells. Growth factor receptors, steroid hormone receptors and signal proteins are among its substrates, so-called client proteins; many of them being targets for anti-cancer therapy. Adverse conditions of the tumor microenvironment, such as hypoxia and nutrient deficiency, contribute to destabilization of proteins and further escalate dependence on chaperones. This is why molecular chaperones, in particular Hsp90, may represent a promising target for anticancer therapy. Importantly also, tumour-based Hsp90 has a significantly higher sensitivity to inhibitors than that in normal cells, and Hsp90 activity inhibition in tumours leads to a suppression of cellular signaling in many different oncogenic pathways. Several inhibitors of Hsp90 are currently undergoing clinical evaluation and new agents with different mechanisms of action are continually being identified.
Key words:
molecular chaperones – Hsp90 – cancer – therapy
This work was supported by grants of MZCR NS/9812-4 and RECAMO CZ.1.05/2.1.00/03.0101.
The Editorial Board declares that the manuscript met the ICMJE “uniform requirements” for biomedical papers.
Submitted:
18. 4. 2011
Accepted:
25. 5. 2011
Sources
1. Hartl FU, Hayer-Hartl M. Converging concepts of protein folding in vitro and in vivo. Nat Struct Mol Biol 2009; 16(6): 574–581.
2. Taipale M, Jarosz DF, Lindquist S. HSP90 at the hub of protein homeostasis: emerging mechanistic insights. Nat Rev Mol Cell Biol 2010; 11(7): 515–528.
3. Horwich AL, Apetri AC, Fenton WA. The GroEL/GroES cis cavity as a passive anti-aggregation device. FEBS Lett 2009; 583(16): 2654–2662.
4. Wegele H, Müller L, Buchner J. Hsp70 and Hsp90 – a relay team for protein folding. Rev Physiol Biochem Pharmacol 2004; 151: 1–44.
5. Nakamoto H, Vigh L. The small heat shock proteins and their clients. Cell Mol Life Sci 2007; 64(3): 294–306.
6. Laskey RA, Honda BM, Mills AD et al. Nucleosomes are assembled by an acidic protein which binds histones and transfers them to DNA. Nature 1978; 275(5679): 416–420.
7. Besche HC, Haas W, Gygi SP et al. Isolation of mammalian 26S proteasomes and p97/VCP complexes using the ubiquitin-like domain from HHR23B reveals novel proteasome-associated proteins. Biochemistry 2009; 48(11): 2538–2549.
8. Brugge JS, Erikson E, Erikson RL. The specific interaction of the Rous sarcoma virus transforming protein, pp60src, with two cellular proteins. Cell 1981; 25(2): 363–372.
9. Sreedhar AS, Kalmár E, Csermely P et al. Hsp90 isoforms: functions, expression and clinical importance. FEBS Lett 2004; 562(1–3): 11–15.
10. Tsutsumi S, Neckers L. Extracellular heat shock protein 90: a role for a molecular chaperone in cell motility and cancer metastasis. Cancer Sci 2007; 98(10): 1536–1539.
11. Eustace BK, Sakurai T, Stewart JK et al. Functional proteomic screens reveal an essential extracellular role for hsp90 alpha in cancer cell invasiveness. Nat Cell Biol 2004; 6(6): 507–514.
12. Dutta R, Inouye M. GHKL, an emergent ATPase/kinase superfamily. Trends Biochem Sci 2000; 25(1): 24–28.
13. Hainzl O, Lapina MC, Buchner J et al. The charged linker region is an important regulator of Hsp90 function. J Biol Chem 2009; 284(34): 22559–22567.
14. Hawle P, Siepmann M, Harst A et al. The middle domain of Hsp90 acts as a discriminator between different types of client proteins. Mol Cell Biol 2006; 26(22): 8385–5395.
15. Minami Y, Kimura Y, Kawasaki H et al. The carboxy-terminal region of mammalian HSP90 is required for its dimerization and function in vivo. Mol Cell Biol 1994; 14(2): 1459–1464.
16. Young JC, Obermann WM, Hartl FU. Specific binding of tetratricopeptide repeat proteins to the C-terminal 12-kDa domain of hsp90. J Biol Chem 1998; 273(29): 18007–18010.
17. Prodromou C, Panaretou B, Chohan S et al. The ATPase cycle of Hsp90 drives a molecular ‚clamp‘ via transient dimerization of the N-terminal domains. EMBO J 2000; 19(16): 4383–4392.
18. Panaretou B, Siligardi G, Meyer P et al. Activation of the ATPase activity of hsp90 by the stress-regulated cochaperone aha1. Mol Cell 2002; 10(6): 1307–1318.
19. Richter K, Walter S, Buchner J. The Co-chaperone Sba1 connects the ATPase reaction of Hsp90 to the progression of the chaperone cycle. J Mol Biol 2004; 342(5): 1403–1413.
20. Lee P, Shabbir A, Cardozo C et al. Sti1 and Cdc37 can stabilize Hsp90 in chaperone complexes with a protein kinase. Mol Biol Cell 2004; 15(4): 1785–1792.
21. Roe SM, Ali MM, Meyer P et al. The Mechanism of Hsp90 regulation by the protein kinase-specific cochaperone p50(cdc37). Cell 2004; 116(1): 87–98.
22. Wandinger SK, Richter K, Buchner J. The Hsp90 chaperone machinery. J Biol Chem 2008; 283(27): 18473–18477.
23. Workman P. Altered states: selectively drugging the Hsp90 cancer chaperone. Trends Mol Med 2004; 10(2): 47–51.
24. Hanahan D, Weinberg RA. The hallmarks of cancer. Cell 2000; 100(1): 57–70.
25. Kitano H. Cancer robustness: tumour tactics. Nature 2003; 426(6963): 125.
26. Kobayashi S, Boggon TJ, Dayaram T et al. EGFR mutation and resistance of non-small-cell lung cancer to gefitinib. N Engl J Med 2005; 352(8): 786–792.
27. Pashtan I, Tsutsumi S, Wang S et al. Targeting Hsp90 prevents escape of breast cancer cells from tyrosine kinase inhibition. Cell Cycle 2008; 7(18): 2936–2941.
28. Sangster TA, Lindquist S, Queitsch C. Under cover: causes, effects and implications of Hsp90-mediated genetic capacitance. Bioessays 2004; 26(4): 348–362.
29. Gatenby RA, Maini PK. Mathematical oncology: cancer summed up. Nature 2003; 421(6921): 321.
30. Whitesell L, Lindquist SL. HSP90 and the chaperoning of cancer. Nat Rev Cancer 2005; 5(10): 761–772.
31. Whitesell L, Mimnaugh EG, De Costa B et al. Inhibition of heat shock protein HSP90-pp60v-src heteroprotein complex formation by benzoquinone ansamycins: essential role for stress proteins in oncogenic transformation. Proc Natl Acad Sci U S A 1994; 91(18): 8324–8328.
32. Kamal A, Thao L, Sensintaffar J et al. A high-affinity conformation of Hsp90 confers tumour selectivity on Hsp90 inhibitors. Nature 2003; 425(6956): 407–410.
33. Whitesell L, Bagatell R, Falsey R. The stress response: implications for the clinical development of hsp90 inhibitors. Curr Cancer Drug Targets 2003; 3(5): 349–358.
34. Blagg BS, Kerr TD. Hsp90 inhibitors: small molecules that transform the Hsp90 protein folding machinery into a catalyst for protein degradation. Med Res Rev 2006; 26(3): 310–338.
35. DeBoer C, Meulman PA, Wnuk RJ et al. Geldanamycin, a new antibiotic. J Antibiot (Tokyo) 1970; 23(9): 442–447.
36. Supko JG, Hickman RL, Grever MR et al. Preclinical pharmacologic evaluation of geldanamycin as an antitumor agent. Cancer Chemother Pharmacol 1995; 36(4): 305–315.
37. Porter JR, Ge J, Lee J et al. Ansamycin inhibitors of Hsp90: nature’s prototype for anti-chaperone therapy. Curr Top Med Chem 2009; 9(15): 1386–1418.
38. Roe SM, Prodromou C, O’Brien R et al. Structural basis for inhibition of the Hsp90 molecular chaperone by the antitumor antibiotics radicicol and geldanamycin. J Med Chem 1999; 42(2): 260–266.
39. Soga S, Shiotsu Y, Akinaga S et al. Development of radicicol analogues. Curr Cancer Drug Targets 2003; 3(5): 359–369.
40. Chiosis G, Timaul MN, Lucas B et al. A small molecule designed to bind to the adenine nucleotide pocket of Hsp90 causes Her2 degradation and the growth arrest and differentiation of breast cancer cells. Chem Biol 2001; 8(3): 289–299.
41. Taldone T, Chiosis G. Purine-scaffold Hsp90 inhibitors. Curr Top Med Chem 2009; 9(15): 1436–1446.
42. Cheung KM, Matthews TP, James K et al. The identification, synthesis, protein crystal structure and in vitro biochemical evaluation of a new 3,4-diarylpyrazole class of Hsp90 inhibitors. Bioorg Med Chem Lett 2005; 15(14): 3338–3343.
43. Marcu MG, Chadli A, Bouhouche I et al. The heat shock protein 90 antagonist novobiocin interacts with a previously unrecognized ATP-binding domain in the carboxyl terminus of the chaperone. J Biol Chem 2000; 275(47): 37181–37186.
44. Holzbeierlein JM, Windsperger A, Vielhauer G. Hsp90: a drug target? Curr Oncol Rep 2010; 12(2): 95–101.
45. Smith JR, Clarke PA, de Billy E et al. Silencing the cochaperone CDC37 destabilizes kinase clients and sensitizes cancer cells to HSP90 inhibitors. Oncogene 2009; 28(2): 157–169.
46. Westerheide SD, Bosman JD, Mbadugha BN et al. Celastrols as inducers of the heat shock response and cytoprotection. J Biol Chem 2004; 279(53): 56053–56060.
47. Zhang T, Li Y, Yu Y et al. Characterization of celastrol to inhibit hsp90 and cdc37 interaction. J Biol Chem 2009; 284(51): 35381–35389.
48. Kim YS, Alarcon SV, Lee S et al. Update on Hsp90 inhibitors in clinical trial. Curr Top Med Chem 2009; 9(15): 1479–1492.
49. Burris HA 3rd, Berman D, Murthy B et al. Tanespimycin pharmacokinetics: a randomized dose-escalation crossover phase 1 study of two formulations. Cancer Chemother Pharmacol 2011; 67(5): 1045–1054.
50. Chandran T, Katragadda U, Teng Q et al. Design and evaluation of micellar nanocarriers for 17-allyamino-17-demethoxygeldanamycin (17-AAG). Int J Pharm 2010; 392(1–2): 170–177.
51. Xiong MP, Yáñez JA, Kwon GS et al. A cremophor-free formulation for tanespimycin (17-AAG) using PEO-b-PDLLA micelles: characterization and pharmacokinetics in rats. J Pharm Sci 2009; 98(4): 1577–1586.
52. Grem JL, Morrison G, Guo XD et al. Phase I and pharmacologic study of 17-(allylamino)-17-demethoxygeldanamycin in adult patients with solid tumors. J Clin Oncol 2005; 23(9): 1885–1893.
53. Banerji U, O’Donnell A, Scurr M et al. Phase I pharmacokinetic and pharmacodynamic study of 17-allylamino, 17-demethoxygeldanamycin in patients with advanced malignancies. J Clin Oncol 2005; 23(18): 4152–4161.
54. Solit DB, Osman I, Polsky D et al. Phase II trial of 17-allylamino-17-demethoxygeldanamycin in patients with metastatic melanoma. Clin Cancer Res 2008; 14(24): 8302–8307.
55. Ramalingam SS, Egorin MJ, Ramanathan RK et al. A phase I study of 17-allylamino-17-demethoxygeldanamycin combined with paclitaxel in patients with advanced solid malignancies. Clin Cancer Res 2008; 14(11): 3456–3461.
56. Tse AN, Klimstra DS, Gonen M et al. A phase 1 dose-escalation study of irinotecan in combination with 17-allylamino-17-demethoxygeldanamycin in patients with solid tumors. Clin Cancer Res 2008; 14(20): 6704–6711.
57. Hubbard J, Erlichman C, Toft DO et al. Phase I study of 17-allylamino-17 demethoxygeldanamycin, gemcitabine and/or cisplatin in patients with refractory solid tumors. Invest New Drugs 2011; 29(3): 473–480.
58. Richardson PG, Badros AZ, Jagannath S et al. Tanespimycin with bortezomib: activity in relapsed/refractory patients with multiple myeloma. Br J Haematol 2010; 150(4): 428–437.
59. Modi S, Stopeck AT, Gordon MS et al. Combination of trastuzumab and tanespimycin (17-AAG, KOS-953) is safe and active in trastuzumab-refractory HER-2 overexpressing breast cancer: a phase I dose-escalation study. J Clin Oncol 2007; 25(34): 5410–5417.
60. Tsuruo T, Naito M, Tomida A et al. Molecular targeting therapy of cancer: drug resistance, apoptosis and survival signal. Cancer Sci 2003; 94(1): 15–21.
61. Kelland LR, Sharp SY, Rogers PM et al. DT-Diaphorase expression and tumor cell sensitivity to 17-allylamino, 17-demethoxygeldanamycin, an inhibitor of heat shock protein 90. J Natl Cancer Inst 1999; 91(22): 1940–1949.
62. Gaspar N, Sharp SY, Pacey S et al. Acquired resistance to 17-allylamino-17-demethoxygeldanamycin (17-AAG, tanespimycin) in glioblastoma cells. Cancer Res 2009; 69(5): 1966–1975.
63. Kim HR, Kang HS, Kim HD. Geldanamycin induces heat shock protein expression through activation of HSF1 in K562 erythroleukemic cells. IUBMB Life 1999; 48(4): 429–433.
64. McCollum AK, TenEyck CJ, Stensgard B et al. P-Glycoprotein-mediated resistance to Hsp90-directed therapy is eclipsed by the heat shock response. Cancer Res 2008; 68(18): 7419–7427.
65. Ramanathan RK, Egorin MJ, Erlichman C et al. Phase I pharmacokinetic and pharmacodynamic study of 17-dimethylaminoethylamino-17-demethoxygeldanamycin, an inhibitor of heat-shock protein 90, in patients with advanced solid tumors. J Clin Oncol 2010; 28(9): 1520–1526.
66. Kummar S, Gutierrez ME, Gardner ER et al. Phase I trial of 17-dimethylaminoethylamino-17-demethoxygeldanamycin (17-DMAG), a heat shock protein inhibitor, administered twice weekly in patients with advanced malignancies. Eur J Cancer 2010; 46(2): 340–347.
67. Lancet JE, Gojo I, Burton M et al. Phase I study of the heat shock protein 90 inhibitor alvespimycin (KOS-1022, 17-DMAG) administered intravenously twice weekly to patients with acute myeloid leukemia. Leukemia 2010; 24(4): 699–705.
68. Sequist LV, Gettinger S, Senzer NN et al. Activity of IPI-504, a novel heat-shock protein 90 inhibitor, in patients with molecularly defined non-small-cell lung cancer. J Clin Oncol 2010; 28(33): 4953–4960.
Labels
Paediatric clinical oncology Surgery Clinical oncologyArticle was published in
Clinical Oncology
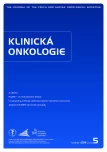
2011 Issue 5
- Metamizole at a Glance and in Practice – Effective Non-Opioid Analgesic for All Ages
- Metamizole vs. Tramadol in Postoperative Analgesia
- Possibilities of Using Metamizole in the Treatment of Acute Primary Headaches
- Current Insights into the Antispasmodic and Analgesic Effects of Metamizole on the Gastrointestinal Tract
- Spasmolytic Effect of Metamizole
Most read in this issue
- Partial Regression of CNS Lesions of Erdheim-Chester Disease after Treatment with 2-chlorodeoxadenosine and Their Full Remission Following Treatment with Lenalidomide
- Neuroendoscopic Biopsy of a Brain Tumor
- Secondary Angiosarcomas after Conservation Treatment for Breast Cancers
- Neoadjuvant Chemoradiotherapy of Rectal Carcinoma with Bevacizumab