Quantitative profiling of genes associated with cancer pathways in brain tumors
Authors:
Z. Majerčíková 1; K. Dibdiaková 1; M. Galanda 2; T. Galanda 2; R. Richterová 3; B. Kolarovszki 3; P. Račay 1; J. Hatok 1
Authors place of work:
Ústav lekárskej biochémie, Jesseniova lekárska fakulta UK v Martine, Martin, Slovenská republika
1; Neurochirurgická klinika SZU, Fakultná nemocnica s poliklinikou F. D. Roosevelta, Banská Bystrica, Slovenská republika
2; Neurochirurgická klinika, Jesseniova lekárska fakulta UK a UN v Martine, Martin, Slovenská republika
3
Published in the journal:
Klin Onkol 2023; 36(3): 224-233
Category:
Original Articles
doi:
https://doi.org/10.48095/ccko2023224
Summary
Background: Brain tumors are a heterogeneous group of malignancies characterized by inter- and intratumoral heterogeneity. Among them, the most aggressive and, despite advances in medicine, still incurable remains glioblastoma. One of the reasons is the high recurrence rate of the disease and resistance to temozolomide, a golden standard in chemotherapy of brain tumors. Therefore, mapping the pathways responsible for tumorigenesis at the transcriptional level may help to determine the causes and aggressive behavior among different glial tumors. Patients and methods: Biopsies from patients with astrocytoma (N = 6), glioblastoma (N = 22), and meningioma (N = 14) were included in the sample set. A control group consisted of RNA isolated from healthy human brain (N = 3). The reverse-transcribed cDNAs were analyzed using the Human Cancer PathwayFinder™ real-time PCR Array in a 96-well format. The expression of 84 genes belonging to 9 signaling pathways (angiogenesis, apoptosis, cell cycle and senescence, DNA damage and repair, epithelial-to-mesenchymal transition, hypoxia, overall metabolism, and telomere dynamics) was determined for each sample. Results: By determining the relative expression of selected genes, we characterized the transcriptomic profile of individual brain malignancies in the context of signaling pathways involved in tumorigenesis. We observed deregulation in 50, 52.4 and 53.6% % of the genes in glioblastomas, meningiomas and astrocytomas, respectively. The most pronounced changes with statistical significance compared to control were observed in the genes associated with epithelial-to-mesenchymal transition (CDH2, FOXC2, GSC, SNAI2, and SOX10), cellular senescence (BMI1, ETS2, MAP2K1, and SOD1), DNA repair (DDB2, ERCC3, GADD45G, and LIG4), and dynamic of telomeres (TEP1, TERF2IP, TNKS, and TNKS2). Conclusion: Based on the obtained data, we can conclude that individual diagnoses differ in transcriptomic profile. An individual molecular approach is therefore necessary in order to provide comprehensive and targeted therapy on multiple metabolic pathways in the diagnosis of brain tumors.
Keywords:
brain tumors – mRNA – profiling of tumorigenesis pathways
Zdroje
1. Louis DN, Perry A, Wesseling P et al. The 2021 WHO classification of tumors of the central nervous system: a summary. Neuro Oncol 2021; 23 (8): 1231–1251. doi: 10.1093/neuonc/noab106.
2. Nørøxe DS, Poulsen HS, Lassen U. Hallmarks of glioblastoma: a systematic review. ESMO Open 2017; 1 (6): e000144. doi: 10.1136/esmoopen-2016-000144.
3. Altieri R, Zenga F, Fontanella MM et al. Glioma surgery: technological advances to achieve a maximal safe resection. Surg Technol Int 2015; 27: 297–302.
4. Agnihotri S, Burrell KE, Wolf A et al. Glioblastoma, a brief review of history, molecular genetics, animal models and novel therapeutic strategies. Arch Immunol Ther Exp (Warsz) 2013; 61 (1): 25–41. doi: 10.1007/s00005-012-0203-0.
5. Wen PY, Kesari S. Malignant gliomas in adults. N Engl J Med 2008; 359 (5): 492–507. doi: 10.1056/NEJMra0708126.
6. Stupp R, Mason WP, van den Bent MJ et al. Radiotherapy plus concomitant and adjuvant temozolomide for glioblastoma. N Engl J Med 2005; 352 (10): 987–996. doi: 10.1056/NEJMoa043330.
7. Blahovcova E, Richterova R, Kolarovszki B et al. Apoptosis-related gene expression in tumor tissue samples obtained from patients diagnosed with glioblastoma multiforme. Int J Mol Med 2015; 36 (6): 1677–1684. doi: 10.3892/ijmm.2015.2369.
8. Narsia N, Ramagiri P, Ehrmann J et al. Transcriptome analysis reveals distinct gene expression profiles in astrocytoma grades II-IV. Biomed Pap Med Fac Univ Palacky Olomouc Czech Repub 2017; 161 (3): 261–271. doi: 10.5507/bp.2017.020.
9. Majercikova Z, Dibdiakova K, Gala M et al. Different approaches for the profiling of cancer pathway-related genes in glioblastoma cells. Int J Mol Sci 2022; 23 (18): 10883. doi: 10.3390/ijms231810883.
10. Hanahan D, Weinberg RA. The hallmarks of cancer. Cell 2000; 100 (1): 57–70. doi: 10.1016/s0092-8674 (00) 81683-9.
11. Takano S, Yamashita T, Ohneda O. Molecular therapeutic targets for glioma angiogenesis. J Oncol 2010; 2010: 351908. doi: 10.1155/2010/351908.
12. Louis DN, Ohgaki H, Wiestler OD et al. The 2007 WHO classification of tumours of the central nervous system. Acta Neuropathol 2007; 114 (2): 97–109. doi: 10.1007/s00401-007-0243-4.
13. Siedlecki Z, Nowak K, Grzyb S et al. Angiogenesis in brain tumors: gliomas and meningiomas – a review. Eur J Mol Clin Med 2021; 8 (2): 2354–2359.
14. Winter RC, Antunes ACM, de Oliveira FH. The relationship between vascular endothelial growth factor and histological grade in intracranial meningioma. Surg Neurol Int 2020; 11: 328. doi: 10.25259/SNI_528_2020.
15. Reszec J, Hermanowicz A, Rutkowski R et al. Expression of MMP-9 and VEGF in meningiomas and their correlation with peritumoral brain edema. Biomed Res Int 2015; 2015: 646853. doi: 10.1155/2015/646853.
16. Loureiro LVM, Neder L, Callegaro-Filho D et al. The immunohistochemical landscape of the VEGF family and its receptors in glioblastomas. Surg Exp Pathol 2020; 3 (9). doi: 10.1186/s42047-020-00060-5.
17. Plate KH, Breier G, Weich HA et al. Vascular endothelial growth factor is a potential tumour angiogenesis factor in human gliomas in vivo. Nature 1992; 359 (6398): 845–848. doi: 10.1038/359845a0.
18. Phillips H, Armani M, Stavrou D et al. Intense focal expression of vascular endothelial growth-factor messenger-RNA in human intracranial neoplasms – association with regions of necrosis. Int J Oncol 1993; 2 (6): 913–919. doi: 10.3892/ijo.2.6.913.
19. Schneider K, Weyerbrock A, Doostkam S et al. Lack of evidence for PlGF mediating the tumor resistance after anti-angiogenic therapy in malignant gliomas. J Neurooncol 2015; 121 (2): 269–278. doi: 10.1007/s11060-014-1647-3.
20. Hess K, Spille DC, Adeli A et al. Occurrence of fibrotic tumor vessels in grade I meningiomas is strongly associated with vessel density, expression of VEGF, PlGF, IGFBP-3 and tumor recurrence. Cancers (Basel) 2020; 12 (10): 3075. doi: 10.3390/cancers12103075.
21. Liao D, Johnson RS. Hypoxia: a key regulator of angiogenesis in cancer. Cancer Metastasis Rev 2007; 26 (2): 281–290. doi: 10.1007/s10555-007-9066-y.
22. Nishie A, Ono M, Shono T et al. Macrophage infiltration and heme oxygenase-1 expression correlate with angiogenesis in human gliomas. Clin Cancer Res 1999; 5 (5): 1107–1113.
23. El Andaloussi A, Lesniak MS. CD4+ CD25+ FoxP3+ T-cell infiltration and heme oxygenase-1 expression correlate with tumor grade in human gliomas. J Neurooncol 2007; 83 (2): 145–152. doi: 10.1007/s11060-006-9314-y.
24. Sferrazzo G, Di Rosa M, Barone E et al. Heme oxygenase-1 in central nervous system malignancies. J Clin Med 2020; 9 (5): 1562. doi: 10.3390/jcm9051562.
25. Ye W, Liu Z, Liu F et al. Heme oxygenase-1 predicts risk stratification and immunotherapy efficacy in lower grade gliomas. Front Cell Dev Biol 2021; 9: 760800. doi: 10.3389/fcell.2021.760800.
26. Airley RE, Mobasheri A. Hypoxic regulation of glucose transport, anaerobic metabolism and angiogenesis in cancer: novel pathways and targets for anticancer therapeutics. Chemotherapy 2007; 53 (4): 233–256. doi: 10.1159/000104457.
27. Pliszka M, Szablewski L. Glucose transporters as a target for anticancer therapy. Cancers (Basel) 2021; 13 (16): 4184. doi: 10.3390/cancers13164184.
28. van de Nes JA, Griewank KG, Schmid KW et al. Immunocytochemical analysis of glucose transporter protein-1 (GLUT-1) in typical, brain invasive, atypical and anaplastic meningioma. Neuropathology 2015; 35 (1): 24–36. doi: 10.1111/neup.12148.
29. Komaki S, Sugita Y, Furuta T et al. Expression of GLUT1 in pseudopalisaded and perivascular tumor cells is an independent prognostic factor for patients with glioblastomas. J Neuropathol Exp Neurol 2019; 78 (5): 389–397. doi: 10.1093/jnen/nly124.
30. Marie SK, Shinjo SM. Metabolism and brain cancer. Clinics (Sao Paulo) 2011; 66 (Suppl 1): 33–43. doi: 10.1590/s1807-59322011001300005.
31. Silva JM, Wippel HH, Santos MDM et al. Proteomics pinpoints alterations in grade I meningiomas of male versus female patients. Sci Rep 2020; 10 (1): 10335. doi: 10.1038/s41598-020-67113-3.
32. Xu W, Yang Z, Lu N. A new role for the PI3K/Akt signaling pathway in the epithelial-mesenchymal transition. Cell Adh Migr 2015; 9 (4): 317–324. doi: 10.1080/19336918.2015.1016686.
33. Johansen MD, Rochat P, Law I et al. Presentation of two cases with early extracranial metastases from glioblastoma and review of the literature. Case Rep Oncol Med 2016; 2016: 8190950. doi: 10.1155/2016/8190950.
34. Chaffer CL, Weinberg RA. A perspective on cancer cell metastasis. Science 2011; 331 (6024): 1559–1564. doi: 10.1126/science.1203543.
35. Armento A, Ehlers J, Schötterl S et al. (2017) Molecular mechanisms of glioma cell motility. In: De Vleeschouwer S (ed). Glioblastoma. Brisbane: Codon Publications 2017: 73–93.
36. Noronha C, Ribeiro AS, Taipa R et al. Cadherin expression and EMT: a focus on gliomas. Biomedicines 2021; 9 (10): 1328. doi: 10.3390/biomedicines9101328.
37. Asano K, Duntsch CD, Zhou Q et al. Correlation of N-cadherin expression in high grade gliomas with tissue invasion. J Neurooncol 2004; 70 (1): 3–15. doi: 10.1023/b: neon.0000040811.14908.f2.
38. Camand E, Peglion F, Osmani N et al. N-cadherin expression level modulates integrin-mediated polarity and strongly impacts on the speed and directionality of glial cell migration. J Cell Sci 2012; 125 (Pt 4): 844–857. doi: 10.1242/jcs.087668.
39. Yang HW, Menon LG, Black PM et al. SNAI2/Slug promotes growth and invasion in human gliomas. BMC Cancer 2010; 10: 301. doi: 10.1186/1471-2407-10-301.
40. Liu B, Dong H, Lin X et al. RND3 promotes Snail 1 protein degradation and inhibits glioblastoma cell migration and invasion. Oncotarget 2016; 7 (50): 82411–82423. doi: 10.18632/oncotarget.12396.
41. Gras B, Jacqueroud L, Wierinckx A et al. Snail family members unequally trigger EMT and thereby differ in their ability to promote the neoplastic transformation of mammary epithelial cells. PLoS One 2014; 9 (3): e92254. doi: 10.1371/journal.pone.0092254.
42. Wang YW, Yin CL, Zhang HY et al. High expression of forkhead box protein C2 is related to poor prognosis in human gliomas. Asian Pac J Cancer Prev 2014; 15 (24): 10621–10625. doi: 10.7314/apjcp.2014.15.24.10621.
43. Wong RS. Apoptosis in cancer: from pathogenesis to treatment. J Exp Clin Cancer Res 2011; 30 (1): 87. doi: 10.1186/1756-9966-30-87.
44. Tirapelli DP, Menezes SB, Franco IM et al. High expression of anti-apoptotic genes in grade I and II meningiomas. Arq Neuropsiquiatr 2017; 75 (4): 209–215. doi: 10.1590/0004-282X20170027.
45. Deveraux QL, Roy N, Stennicke HR et al. IAPs block apoptotic events induced by caspase-8 and cytochrome c by direct inhibition of distinct caspases. EMBO J 1998; 17 (8): 2215–2223. doi: 10.1093/emboj/17.8.2215.
46. Murphy ÁC, Weyhenmeyer B, Schmid J et al. Activation of executioner caspases is a predictor of progression-free survival in glioblastoma patients: a systems medicine approach. Cell Death Dis 2013; 4 (5): e629. doi: 10.1038/cddis.2013.157.
47. Irmler M, Thome M, Hahne M et al. Inhibition of death receptor signals by cellular FLIP. Nature 1997; 388 (6638): 190–195. doi: 10.1038/40657.
48. Sharpless NE, Sherr CJ. Forging a signature of in vivo senescence. Nat Rev Cancer 2015; 15 (7): 397–408. doi: 10.1038/nrc3960.
49. Schuringa JJ, Vellenga E. Role of the polycomb group gene BMI1 in normal and leukemic hematopoietic stem and progenitor cells. Curr Opin Hematol 2010; 17 (4): 294–299. doi: 10.1097/MOH.0b013e328338c439.
50. Molofsky AV, Pardal R, Iwashita T et al. Bmi-1 dependence distinguishes neural stem cell self-renewal from progenitor proliferation. Nature 2003; 425 (6961): 962–967. doi: 10.1038/nature02060.
51. Abdouh M, Facchino S, Chatoo W et al. BMI1 sustains human glioblastoma multiforme stem cell renewal. J Neurosci 2009; 29 (28): 8884–8896. doi: 10.1523/JNEUROSCI.0968-09.2009.
52. Vora P, Seyfrid M, Venugopal C et al. Bmi1 regulates human glioblastoma stem cells through activation of differential gene networks in CD133+ brain tumor initiating cells. J Neurooncol 2019; 143 (3): 417–428. doi: 10.1007/s11060-019-03192-1.
53. Fry EA, Inoue K. Aberrant expression of ETS1 and ETS2 proteins in cancer. Cancer Rep Rev 2018; 2 (3): 10.15761/CRR.1000151. doi: 10.15761/CRR.1000151.
54. Babal YK, Kandemir B, Kurnaz IA. Gene regulatory network of ETS domain transcription factors in different stages of glioma. J Pers Med 2021; 11 (2): 138. doi: 10.3390/jpm11020138.
55. Noor R, Mittal S, Iqbal J. Superoxide dismutase – applications and relevance to human diseases. Med Sci Monit 2002; 8 (9): RA210–RA215.
56. Gao Z, Sarsour EH, Kalen AL et al. Late ROS accumulation and radiosensitivity in SOD1-overexpressing human glioma cells. Free Radic Biol Med 2008; 45 (11): 1501–1509. doi: 10.1016/j.freeradbiomed.2008.08.009.
57. Lu WC, Xie H, Yuan C et al. Identification of potential biomarkers and candidate small molecule drugs in glioblastoma. Cancer Cell Int 2020; 20: 419. doi: 10.1186/s12935-020-01515-1.
58. Zhou Z, Peng B, Li J et al. Integrative pan-cancer analysis of MEK1 aberrations and the potential clinical implications. Sci Rep 2021; 11 (1): 18366. doi: 10.1038/s41598-021-97840-0.
59. Cheaney B 2nd, Bowden S, Krause K et al. An unusual recurrent high-grade glioneuronal tumor with MAP2K1 mutation and CDKN2A/B homozygous deletion. Acta Neuropathol Commun 2019; 7 (1): 110. doi: 10.1186/s40478-019-0763-x.
60. Gousias K, Theocharous T, Simon M. Mechanisms of cell cycle arrest and apoptosis in glioblastoma. Biomedicines 2022; 10 (3): 564. doi: 10.3390/biomedicines10030564.
61. Ozon S, Maucuer A, Sobel A. The stathmin family – molecular and biological characterization of novel mammalian proteins expressed in the nervous system. Eur J Biochem 1997; 248 (3): 794–806. doi: 10.1111/j.1432-1033.1997.t01-2-00794.x.
62. Devred F, Tsvetkov PO, Barbier P et al. Stathmin/Op18 is a novel mediator of vinblastine activity. FEBS Lett 2008; 582 (17): 2484–2488. doi: 10.1016/j.febslet.2008.06.035.
63. Howitt BE, Nucci MR, Drapkin R et al. Stathmin-1 expression as a complement to p16 helps identify high- -grade cervical intraepithelial neoplasia with increased specificity. Am J Surg Pathol 2013; 37 (1): 89–97. doi: 10.1097/PAS.0b013e3182753f5a.
64. Hemdan T, Lindén M, Lind SB et al. The prognostic value and therapeutic target role of stathmin-1 in urinary bladder cancer. Br J Cancer 2014; 111 (6): 1180–1187. doi: 10.1038/bjc.2014.427.
65. Sun R, Liu Z, Wang L et al. Overexpression of stathmin is resistant to paclitaxel treatment in patients with non--small cell lung cancer. Tumour Biol 2015; 36 (9): 7195–7204. doi: 10.1007/s13277-015-3361-y.
66. Dong B, Mu L, Qin X et al. Stathmin expression in glioma-derived microvascular endothelial cells: a novel therapeutic target. Oncol Rep 2012; 27 (3): 714–718. doi: 10.3892/or.2011.1525.
67. Wang H, Li W, Wang G et al. Overexpression of STMN1 is associated with the prognosis of meningioma patients. Neurosci Lett 2017; 654: 1–5. doi: 10.1016/j.neulet.2017.06.020.
68. Ghelli Luserna di Rorà A, Cerchione C, Martinelli G et al. A WEE1 family business: regulation of mitosis, cancer progression, and therapeutic target. J Hematol Oncol 2020; 13 (1): 126. doi: 10.1186/s13045-020-00959-2.
69. Magnussen GI, Holm R, Emilsen E et al. High expression of Wee1 is associated with poor disease-free survival in malignant melanoma: potential for targeted therapy. PLoS One 2012; 7 (6): e38254. doi: 10.1371/journal.pone.0038254.
70. Slipicevic A, Holth A, Hellesylt E et al. Wee1 is a novel independent prognostic marker of poor survival in post-chemotherapy ovarian carcinoma effusions. Gynecol Oncol 2014; 135 (1): 118–124. doi: 10.1016/j.ygyno.2014.07.102.
71. Lescarbeau RS, Lei L, Bakken KK et al. Quantitative phosphoproteomics reveals Wee1 kinase as a therapeutic target in a model of proneural glioblastoma. Mol Cancer Ther 2016; 15 (6): 1332–1343. doi: 10.1158/1535-7163.MCT-15-0692.
72. Tashnizi AH, Jaberipour M, Razmkhah M et al. Tumour suppressive effects of WEE1 gene silencing in neuroblastomas. J Cancer Res Ther 2016; 12 (1): 221–227. doi: 10.4103/0973-1482.165861.
73. Griffith JD, Comeau L, Rosenfield S et al. Mammalian telomeres end in a large duplex loop. Cell 1999; 97 (4): 503–514. doi: 10.1016/s0092-8674 (00) 80760-6.
74. Wang C, Meier UT. Architecture and assembly of mammalian H/ACA small nucleolar and telomerase ribonucleoproteins. EMBO J 2004; 23 (8): 1857–1867. doi: 10.1038/sj.emboj.7600181.
75. Shervington A, Patel R, Lu C et al. Telomerase subunits expression variation between biopsy samples and cell lines derived from malignant glioma. Brain Res 2007; 1134 (1): 45–52. doi: 10.1016/j.brainres.2006.11.093.
76. Canudas S, Houghtaling BR, Kim JY et al. Protein requirements for sister telomere association in human cells. EMBO J 2007; 26 (23): 4867–4878. doi: 10.1038/sj.emboj.7601903.
77. Clevers H, Loh KM, Nusse R. Stem cell signaling. An integral program for tissue renewal and regeneration: wnt signaling and stem cell control. Science 2014; 346 (6205): 1248012. doi: 10.1126/science.1248012.
78. Wood RD, Mitchell M, Sgouros J et al. Human DNA repair genes. Science 2001; 291 (5507): 1284–1289. doi: 10.1126/science.1056154.
79. Dizdaroglu M. Oxidatively induced DNA damage and its repair in cancer. Mutat Res Rev Mutat Res 2015; 763: 212–245. doi: 10.1016/j.mrrev.2014.11.002.
80. Zhao R, Cui T, Han C et al. DDB2 modulates TGF-b signal transduction in human ovarian cancer cells by downregulating NEDD4L. Nucleic Acids Res 2015; 43 (16): 7838–7849. doi: 10.1093/nar/gkv667.
81. Batista LF, Roos WP, Christmann M et al. Differential sensitivity of malignant glioma cells to methylating and chloroethylating anticancer drugs: p53 determines the switch by regulating xpc, ddb2, and DNA double-strand breaks. Cancer Res 2007; 67 (24): 11886–11895. doi: 10.1158/0008-5472.CAN-07-2964.
82. Kun S, Duan Q, Liu G et al. Prognostic value of DNA repair genes based on stratification of glioblastomas. Oncotarget 2017; 8 (35): 58222–58230. doi: 10.18632/oncotarget.17452.
83. Hanahan D, Weinberg RA. Hallmarks of cancer: the next generation. Cell 2011; 144 (5): 646–674. doi: 10.1016/j.cell.2011.02.013.
84. Soupene E, Kuypers FA. Mammalian long-chain acyl-CoA synthetases. Exp Biol Med (Maywood) 2008; 233 (5): 507–521. doi: 10.3181/0710-MR-287.
85. Lopes-Marques M, Cunha I, Reis-Henriques MA et al. Diversity and history of the long-chain acyl-CoA synthetase (Acsl) gene family in vertebrates. BMC Evol Biol 2013; 13: 271. doi: 10.1186/1471-2148-13-271.
86. Chen WC, Wang CY, Hung YH et al. Systematic analysis of gene expression alterations and clinical outcomes for long-chain acyl-coenzyme A synthetase family in cancer. PLoS One 2016; 11 (5): e0155660. doi: 10.1371/journal.pone.0155660.
87. Cheng J, Fan YQ, Liu BH et al. ACSL4 suppresses glioma cells proliferation via activating ferroptosis. Oncol Rep 2020; 43 (1): 147–158. doi: 10.3892/or.2019.7419.
88. Sanzey M, Abdul Rahim SA, Oudin A et al. Comprehensive analysis of glycolytic enzymes as therapeutic targets in the treatment of glioblastoma. PLoS One 2015; 10 (5): e0123544. doi: 10.1371/journal.pone.0123544.
89. Stanke KM, Wilson C, Kidambi S. High expression of glycolytic genes in clinical glioblastoma patients correlates with lower survival. Front Mol Biosci 2021; 8: 752404. doi: 10.3389/fmolb.2021.752404.
90. Bai X, Tan TY, Li YX et al. The protective effect of cordyceps sinensis extract on cerebral ischemic injury via modulating the mitochondrial respiratory chain and inhibiting the mitochondrial apoptotic pathway. Biomed Pharmacother 2020; 124: 109834. doi: 10.1016/j.biopha.2020.109834.
Štítky
Paediatric clinical oncology Surgery Clinical oncologyČlánok vyšiel v časopise
Clinical Oncology
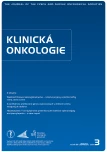
2023 Číslo 3
- Metamizole at a Glance and in Practice – Effective Non-Opioid Analgesic for All Ages
- Current Insights into the Antispasmodic and Analgesic Effects of Metamizole on the Gastrointestinal Tract
- Obstacle Called Vasospasm: Which Solution Is Most Effective in Microsurgery and How to Pharmacologically Assist It?
- Spasmolytic Effect of Metamizole
- Metamizole in perioperative treatment in children under 14 years – results of a questionnaire survey from practice
Najčítanejšie v tomto čísle
- Waldenström’s macroglobulinemia – clinical symptoms and review of therapy yesterday, today and tomorrow
- Polygenic risk score (PRS) and its potential for breast cancer risk stratification
- Molecular testing of endometrial carcinoma in real-world clinical practice
- Hypoplastic form of myelodysplastic neoplasm