Short‑term Effects of Botulinum Toxin A and Serial Casting on Triceps Surae Muscle Length and Equinus Gait in Children with Cerebral Palsy
Vliv léčby botulinum toxinem‑ A a redresního sádrování na délku musculus triceps surae a ekvinózní postavení nohy během chůze u pacientů s dětskou mozkovou obrnou
Cíle:
Cílem této studie bylo provést komplexní analýzu krátkodobého účinku vysokodávkovaného botulinum toxinu- A (BTX‑ A) aplikovaného společně s redresním sádrováním z důvodu ekvinózního postavení nohy během chůze u pacientů s dětskou mozkovou obrnou (DMO). Dalším cílem bylo zhodnotit vliv této léčby na délku musculus triceps surae.
Materiál a metodika:
Jedná se o prospektivní studii, do které bylo zařazeno 10 pacientů (17 končetin) se spastickou formou DMO a ekvinózním postavením nohy. K hodnocení byla použita metoda trojdimenzionální kinematické a kinetické analýzy chůze společně s počítačovou modelací délky musculus triceps surae. Pacienti byli vyšetřeni před a 10 týdnů po léčbě. Ke statistické analýze byl použit párový t- test.
Výsledky:
Rychlost chůze a délka kroku se po aplikaci BTX‑ A nezměnila. Maximální dorziflexe na konci fáze jedné opory se zvýšila (p = 0,045), což vedlo k normalizaci „slope quotient“ (p = 0,001). Došlo také k odstranění patologické generace síly během první poloviny fáze jedné opory (p = 0,012). Oproti očekávání, síla plantiflexorů nebyla aplikací BTX‑ A ovlivněna (p = 0,486). Neprokázali jsme prodloužení musculus triceps surae.
Závěr:
Aplikace BTX‑ A společně s redresním sádrováním vede ke zlepšení kinematiky a kinetiky chůze pacientů s ekvinózní deformitou a DMO. Výsledky naší studie upozorňují na možnost rozdílného působení BTX‑ A na intrafuzální a extrafuzální svalová vlákna.
Klíčová slova:
dětská mozková obrna – botulinum toxin-A – pes equinus – analýza chůze
Authors:
E. B. Zwick 1; M. Švehlík 1,2; G. Steinwender 1; W. E. Linhart 1
Authors place of work:
Czech Republic
; Austria
; Department of Paediatric Orthopaedics, Department of Paediatric Surgery, Medical University of Graz
1; Department of Child and Adult Orthopaedics and Traumatology, 2nd Medical School, Charles University Prague
2
Published in the journal:
Cesk Slov Neurol N 2009; 72/105(6): 553-558
Category:
Original Paper
Summary
Aims:
The aim of the study was to perform a comprehensive analysis of the short‑term effects of a standardized high dose of BTX‑ A and serial casting on spastic equinus in children with cerebral palsy. A second goal was to evaluate this short‑term effect on muscle lengths of the triceps surae.
Materials and methodology:
Prospective study on 10 children (17 limbs) with spastic cerebral palsy and equinus gait pattern. Three- dimensional kinematic and kinetic gait analysis with musclulo- skeletal modelling was used to evaluate pre‑ and 10 weeks post‑treatment results. The paired Student’s T‑test was used for data analysis.
Results:
Walking velocity and stride length did not change after BTX‑ A application. The maximal ankle angle at the end of single support increased (p = 0.045) which led to a change of the ankle angle slope quotient towards normal positive values (p = 0.001). Abnormal ankle power generation in the first part of single stance changed towards normal (p = 0.012). Notably, the ankle plantar- flexor power was not reduced 10 weeks after BTX‑ A injection (p = 0.486). No lengthening of the triceps surae muscles compared to the baseline assessment was found.
Conclusions:
Beneficial functional changes in ankle kinematics and kinetics after the application of BTX‑ A when combined with serial casting were demonstrated. The results of our study underline the possible differences in effect of BTX‑ A on intrafusal and extrafusal muscle fibres.
Key words:
cerebral palsy – botulinum toxin A – pes equinus – gait analysis
Introduction
Cerebral palsy refers to a group of disorders in the development of posture and motor control, occurring as the result of a non‑progressive lesion of the developing central nervous system [1]. Children with cerebral palsy may display a range of movement disorders, alone or in combination, including dystonia, athetosis, ataxia and spasticity [2]. Equinus gait is one of the most common motor manifestations of muscle spasticity in cerebral palsy [3]. Equinus decreases stability during the stance phase of gait, interferes with the smooth translation of the body over the foot and leads to inadequate clearance of the foot in the swing phase. [4]. If untreated, protracted spasticity can lead to fixed muscle contractures that may require surgical intervention.
Previous reports have found that the presence of spasticity increases muscle stiffness and thereby inhibits muscle growth [4,5]. Besides conservative therapies such as physiotherapy, serial casting, orthotics and oral medication, a variety of invasive treatment methods are now commonly available to manage spasticity in cerebral palsy. These include selective dorsal rhizotomy (SDR), intrathecal administration of baclofen (ITB) or botulinum toxin type A (BTX‑A) injections, which has been used clinically for more than 20 years. BTX‑A induces muscle weakness by preventing the release of acetylcholine from the presynaptic axon at the motor endplate [5]. The degree of weakening depends on the dose of BTX‑A and on the number of synapses affected [6]. Spasticity reduction due to the effects of BTX‑A injections typically lasts from 12 to 16 weeks. Re‑innervation takes place by sprouting of new nerve terminals, a process that peaks at 60 days in humans [7]. Functional benefits may last for up to six months or even longer [8]. In addition to the effect of muscle weakening, BTX‑A has been shown to influence the longitudinal growth of an injected muscle in an animal model [9]. Based on computer simulation and modelling, the same effect was confirmed in humans four weeks after BTX‑A application [10].
The motivation for the present study was to perform a comprehensive analysis of the short‑term effects of a standardized dose of BTX‑A and serial casting on spastic equinus in children with cerebral palsy. A second goal was to evaluate this short‑term treatment on muscle length of the triceps surae. To evaluate functional outcome and changes in muscle length, we applied three-dimensional gait analysis and calculated individual dynamic peak muscle lengths by using musculo-skeletal modelling techniques.
Methods
Design
The study is a prospective analysis of the short‑term effects of a standardized high dose of BTX‑A (7 IU/kg) applied to the gastro‑soleus complex and serial casting. The patients were examined before and 10.1 weeks (SD 1.3) after a BTX‑A application. The pre‑treatment gait analysis was used as a baseline for validation of treatment effect.
Subjects
For this investigation, equinus gait was defined as a peak ankle dorsal-flexion of more than one standard deviation below the normal average during the stance phase of gait [11]. Inclusion and exclusion criteria are listed in Table 1. Based on these criteria, 10 children with spastic cerebral palsy were included in the trial (4 males, 6 females). The average age of our study group was 7 years and one month (SD 2 years and 3 months). Seven children were diplegic and three hemiplegic. A total of 17 lower extremities were analysed and used for statistical analysis. The ethics committee of the local medical university approved the study.
Intervention
BTX‑A injections were performed under general anaesthesia. A BTX‑A dose of 7 IU/kg (Botox©, Allergan Inc, Irvine, CA, USA) was applied to multiple sites over the muscle belly of the gastrocnemius and soleus. A multiple injection site technique was applied in which every site was injected with 10 IU BTX‑A. The positioning of the needle in the muscle was as described by Baker et al [10]. A few hours after the BTX‑A injections a below-knee walking cast was applied in maximal ankle dorsal-flexion for a duration of three weeks. Casts were changed every week. Special physiotherapy training was performed after the removal of the third cast in all cases, on an out‑patient basis two times a week for the whole period of the study. The physiotherapy included passive muscle stretching and gait improvement training; either the Vojta concept or the Bobath concept was utilized.
Gait analysis
Computerized gait analysis was performed using a six‑camera, video‑based motion-capturing system (Vicon MX, Oxford Metrics, Oxford, U.K.) and four floor-mounted force plates (Kistler Instruments Limited, Winterthur, Switzerland). Thirteen reflective markers were used to define the pelvis and lower extremity segments in accordance with the Vicon Clinical Manager model (Oxford Metrics, Oxford, U.K.). Moment and power parameters were normalized to the weight of the patients. Power generation and absorption patterns in the sagittal plane were calculated and labelled according to the method described by Winter [12]. All patients walked at self-selected speeds along a 12-metre walkway. For each patient a minimum of five trials, providing clear foot‑force plate contact, were captured and averaged. To better describe the different patterns of ankle kinematics during the single stance phase, a “slope quotient” was employed [13]. The slope quotient of the sagittal plane ankle angle during walking was computed as a subtraction of the ankle angle at the beginning of single support from the ankle angle at the end of the single support. Positive values of the slope quotient indicate muscle elongation, negative values indicate muscle shortening and values close to zero show that a muscle has stayed at the same length during the single support phase of the gait cycle.
Muscle length calculation
Based on kinematic data, Mobile GaitLab software (Duisburg-Essen University, Institute of Mechatronics and System Dynamics, Germany) [14] was used to compute individual muscle lengths of the gastrocnemius and soleus. The specification of the lower extremity architecture was based on the origin and insertion coordinate data compiled by Yamaguchi [15]. The model defines the musculoskeletal geometry of a normal adult male. Three-dimensional musculo-tendon (MT) pathways were modelled as a series of straight‑line segments extending from origin to insertion. Where appropriate, the MT pathways were sub‑sectioned into a number of straight‑line segments by introducing via-points. Musculo-tendon length parameters were normalized to allow comparison between subjects.
Statistics
Ankle and knee kinematic and kinetics in the sagittal plane as well as maximal length of the medial gastrocnemius (MGAC), lateral gastrocnemius (LGAC) and the soleus (SOL) muscles over the gait cycle were considered as main outcome measures for this study. Student’s T-test (Statistica 6.0, StatSoft, Tulsa, OK) was used to describe the pre‑ and post‑treatment differences.
Results
The walking velocity and the stride length did not change after the BTX‑A application. Ankle kinematics and kinetics changed towards normal 10 weeks after the treatment (Fig. 1). The maximal ankle angle at the end of single support increased (p = 0.045) and also the timing of the maximal angle shifted towards normal values (Table 2). The ankle angle slope quotient changed to normal positive values (p = 0.001). We did not observe any changes in knee kinematics. While the maximum ankle moment values remained unchanged, the abnormal ankle moment peak in the first part of the single support phase decreased and the timing of maximum ankle moment occurred later in the stance phase (p = 0.024). An abnormal power generation in the first part of the single support stance phase decreased markedly (p = 0.012). Musculo-skeletal modelling revealed no lengthening of the triceps surae muscles compared to the baseline assessment (Table 2). We did not observe any adverse effects in our study group.
Discussion
This study utilised three-dimensional gait analyses to evaluate the effects of BTX‑A and serial casting on kinematic and kinetic gait parameters in children with cerebral palsy who exhibit equinus gait. Short‑term effects were analysed ten weeks after BTX‑A injections. To evaluate changes in dynamic muscle length, musclulo-skeletal modelling techniques were applied. The results of this study confirm previously reported beneficial short‑term outcome when equinus gait is treated with BTX‑A and serial casting in children with cerebral palsy. Like other authors [7,16] we observed significant improvements of ankle kinematics and kinetics. These improvements include a reduction in equinus and a change of timing and modulation of ankle motion patterns towards normal. During the “second rocker” of ankle motion we documented improved dorsal flexion at the end of the stance phase of the gait cycle [4]. As it has been demonstrated that equinus gait increases the energy expenditure during walking in children with cerebral palsy [17], the improvement of the ankle kinematics might allow for longer walking distances, which could improve the quality of life. The changes in ankle moments and powers observed in this study provide insight into the underlying patho-physiology of the gastro‑soleus muscle in spastic equinus gait. Abnormal power absorption was observed whenever a forefoot landing occurred and the ankle was loaded through a forefoot lever. This power absorption can be interpreted as a calf muscle stretch, which is followed by a burst of power generation in the first half of single support. This burst of power generation points towards a spasticity‑driven stretch reflex after loading the ankle via a forefoot lever arm. When treated with BTX‑A and serial casting the reflex‑induced power generation was markedly reduced. In this study, musculo-skeletal modelling revealed no changes in muscle length that could be attributed to the interventions of BTX‑A and serial casting. This finding contrasts with previous reports [10,18,19] that documented changes in calf muscle length as a beneficial short‑term outcome. As the changes in kinematics and kinetics cannot be attributed to changes in muscle length, possibly BTX‑A affects intrafusal muscle spindles, reducing the effects of the gamma-loop stretch reflex. In a recent study of the long‑term effects of BTX‑A treatment on muscle tone and range of motion in a group of children with cerebral palsy, Tedroff et al [20] demonstrated that BTX‑A was efficient in reducing the muscle tone but the improvement of range of motion lasted for only a short period of time in the gastrocnemius muscle. This evidence would support our conclusion that during the first weeks after the application of BTX‑A, the therapy might have a more significant influence on muscle spasticity than on muscle growth. On the other hand, Kanovsky et al showed a lack of deterioration in maximum passive ankle dorsiflexion for both groups in a paper concerning the long‑term efficacy and tolerability of 4-monthly versus yearly BTX‑A treatment for lower limb spasticity in children with cerebral palsy [21].
The dose of BTX‑A used in this trial (7 IU/kg of BTX‑A for the triceps surae complex) is rather high when compared in the literature [22,23]. However, a randomized, double‑blinded trial demonstrated that high‑dose BTX‑A treatment has a better effect on gait and spasticity improvement than low‑dose BTX‑A treatment [24]. Similar dosages of BTX‑A have been evaluated by other authors [7,25] who did not observe any increase in adverse effects. There is no general agreement on the use of serial casting together with BTX‑A treatment in children with cerebral palsy [26]. It has been shown that serial casting enhanced and prolonged the effect of BTX‑A treatment [7,16]. In a more recent study, Newman et al [27] showed additional benefits in the recurrence of spasticity when casting is delayed after BTX‑A injections. Based on these supportive data and our personal experience, we adopted this procedure for routine use. The cast is not applied immediately after BTX‑A administration but on the same day (approximately four to five hours after injecting BTX‑A). This time is used to move and stretch the triceps surae muscle. It has been proved in an animal model that as few as 10 passive stretch/relax cycles can increase the efficacy of BTX‑A [28]. However, to the best of our knowledge, no published data confirms this finding in a group of children with cerebral palsy. On the other hand, electrical stimulation has been proved to prolong gait improvement in children with spastic diplegic cerebral palsy [29].
Management of cerebral palsy is primarily directed towards maintaining or regaining muscle length. Use of BTX‑A here is based on the concept that muscle fibres in children with spastic cerebral palsy are short, and weakening the muscles with BTX‑A injections might allow them to be stretched and thus grow in length. Thus the progression from dynamic to fixed deformity may be delayed [30] and any orthopaedic surgical procedures might be postponed or reduced in frequency [31]. Although muscle growth following BTX‑A injection has also been found in animal models [9,32], the findings of our study did not support this in children with cerebral palsy in a short‑term follow up. As there is no direct way to measure muscle length in humans, muscle length calculations are based on mathematical models of the lower extremities and on measurements of ankle, knee, hip and pelvis kinematics. It is obvious that such mathematical modelling approaches have limitations, but the method is well accepted in the literature [25,33] because it provides additional information which can help us to better understand the biomechanics of a spastic muscle. We speculate that a period of ten weeks is too short to expect any measurable gain in muscle length by muscle growth. However, we observed significant improvements in ankle kinematics and kinetics. We interpret the beneficial changes in kinematics and kinetics observed in this study as an effect of BTX‑A on intrafusal muscle fibres [34]. Therefore, the application of BTX‑A may have a more significant influence on muscle spasticity than on muscle growth. The BTX‑A is effective in reducing the over‑activity of the spastic muscle and improving its function [35]. Moreover, even if the main effect of BTX‑A might be to weaken the muscle, we observed no decrease in power generation in terminal stance, which is the most important factor for forward propulsion in walking. Again, this could be explained by different influences of BTX‑A on intrafusal and extrafusal muscle fibres. Such differences in outcome might be attributed to the variety of methods used to calculate muscle lengths in the various studies.
In conclusion, this study demonstrated beneficial functional changes in ankle kinematics and kinetics after the application of BTX‑A when combined with serial casting. We did not observe any change in the peak muscle-tendon length of the gastro‑soleus muscle complex. Further studies are needed to address the question of muscle growth after BTX‑A application in children with spastic cerebral palsy.
MUDr. Martin Švehlík
Department of Paediatric Orthopaedics
Department of Paediatric Surgery, Medical University of Graz
Auenbruggerplatz 34
A-8036 Graz
Austria
e-mail: martin.spejlik@seznam.cz
Accepted for review: 15. 7. 2009
Accepted for publication: 21. 8. 2009
Zdroje
1. Bax MC. Terminology and classification of cerebral palsy. Dev Med Child Neurol 1964; 6: 295– 297.
2. Koman LA, Smith BP, Shilt JS. Cerebral palsy. Lancet 2004; 363(9421): 1619– 1631.
3. Gaines RW, Ford TB. A systematic approach to the amount of Achilles tendon lengthening in cerebral palsy. 1984; 4(4): 448– 451.
4. Perry J, Hoffer MM, Giovan P, Antonelli D, Greenberg R. Gait analysis of the triceps surae in cerebral palsy. A preoperative and postoperative clinical and electromyographic study. J Bone Joint Surg Am 1974; 56(3): 511– 520.
5. Kita M, Goodkin DE. Drugs used to treat spasticity. Drugs 2000; 59(3): 487– 495.
6. Spitzer C, Kessler C, Freyberger HJ. Only a gait disorder? A case report of dissociative disorders. Psychiatr Prax 1997; 24(3):150– 151.
7. Corry IS, Cosgrove AP, Duffy CM, McNeill S, Taylor TC, Graham HK. Botulinum toxin. A compared with stretching casts in the treatment of spastic equinus: a randomised prospective trial. J Pediatr Orthop 1998; 18(3): 304– 311.
8. Jefferson RJ. Botulinum toxin in the management of cerebral palsy. Dev Med Child Neurol 2004; 46(7): 491– 499.
9. Ziv I, Blackburn N, Rang M, Koreska J. Muscle growth in normal and spastic mice. Dev Med Child Neurol 1984; 26(1): 94– 99.
10. Baker R, Jasinski M, Maciaq‑ Tymecka I, Michalowska- Mrozek J, Bonikowski M, Carr L et al. Botulinum toxin treatment of spasticity in diplegic cerebral palsy: a randomized, double‑blind, placebo- controlled, dose‑ranging study. Dev Med Child Neurol 2002; 44(10): 666– 675.
11. Kay RM, Rethlefsen SA, Fern- Buneo A, Wren TA, Skaggs DL. Botulinum toxin as an adjunct to serial casting treatment in children with cerebral palsy. J Bone Joint Surg Am 2004; 86(11): 2377– 2384.
12. Winter DA. Energy generation and absorption at the ankle and knee during fast, natural, and slow cadences. Clin Orthop Relat Res 1983; 175:147– 154.
13. Pierce LA, Buckon CE, Sienko TS, Orendurff BS, Piatt JH, Sussman MD et al. Is the double bump ankle moment pattern caused by spasticity. Gait and Posture 1997; 5: 139.
14. Stolz M. Modellbildung, Simulation und Analyse der menschlichen Beinbewegung zur Vorbereitung chirurgischer Eingriffe. Technische Universität, Graz: Unpublished Diplomarbeit 2002.
15. Yamaguchi GT. Dynamic Modeling of Musculoskeletal Motion – A Vectorized Approach for Biomechanical Analysis in Three Dimensions. Norwell, Massachusetts, USA: Kluwer Academic Publishers 2001.
16. Desloovere K, Molenaers G, Jonkers I, De Cat J, De Borre L, Nijs J et al. A randomized study of combined botulinum toxin type A and casting in the ambulant child with cerebral palsy using objective outcome measures. Eur J Neurol 2001; 8 (Suppl 5): 75– 87.
17. Bennett BC, Abel MF, Wolovick A, Franklin T, Allaire PE, Kerrigan DC. Center of mass movement and energy transfer during walking in children with cerebral palsy. Arch Phys Med Rehabil 2005; 86(11): 2189– 2194.
18. Bang MS, Chung SG, Kim SB, Kim SJ. Change of dynamic gastrocnemius and soleus muscle length after block of spastic calf muscle in cerebral palsy. Am J Phys Med Rehabil 2002; 81(10): 760– 764.
19. Corry IS, Cosgrove AP, Duffy CM, McNeill S, Taylor TC, Graham HK. Botulinum toxin A compared with stretching casts in the treatment of spastic equinus: a randomised prospective trial. J Pediatr Orthop 1998; 18(3): 304– 311.
20. Tedroff K, Granath F, Forssberg H, Haglund– Akerlind Y. Long‑term effects of botulinum toxin A in children with cerebral palsy. Dev Med Child Neurol 2009; 51(2): 120– 127.
21. Kanovsky P, Bares M, Severa S, Richardson A. Long‑term efficacy and tolerability of 4– monthly versus yearly botulinum toxin type A treatment for lower‑limb spasticity in children with cerebral palsy. Dev Med Child Neurol 2009; 51: 436– 445.
22. Koman LA, Brashear A, Rosenfeld S, Chambers H, Russman B, Rang M et al. Botulinum toxin type a neuromuscular blockade in the treatment of equinus foot deformity in cerebral palsy: a multicenter, open- label clinical trial. Pediatrics 2001; 108(5): 1062– 1071.
23. Sutherland DH, Kaufman KR, Wyatt MP, Chambers HG, Mubarak SJ. Double‑blind study of botulinum A toxin injections into the gastrocnemius muscle in patients with cerebral palsy. Gait Posture 1999; 10(1): 1– 9.
24. Wissel J, Heinen F, Schenkel A, Doll B, Ebersbach G, Müller J et al. Botulinum toxin A in the management of spastic gait disorders in children and young adults with cerebral palsy: a randomized, double‑blind study of “high‑dose” versus “low‑dose” treatment. Neuropediatrics 1999; 30(3): 120– 124.
25. Eames NW, Baker R, Hill N, Graham K, Taylor T, Cosgrove A. The effect of botulinum toxin A on gastrocnemius length: magnitude and duration of response. Dev Med Child Neurol 1999; 41(4): 226– 232.
26. Lannin N, Scheinberg A, Clark K. AACPDM systematic review of the effectiveness of therapy for children with cerebral palsy after botulinum toxin A injections. Dev Med Child Neurol 2006; 48(6): 533– 539.
27. Newman CJ, Kennedy A, Walsh M, O’Brien T, Lynch B, Hensey O. A pilot study of delayed versus immediate serial casting after botulinum toxin injection for partially reducible spastic equinus. J Pediatr Orthop 2007; 27(8): 882– 885.
28. Minamoto VB, Hulst JB, Lim M, Peace WJ, Bremner SN, Ward SR et al. Increased efficacy and decreased systemic- effects of botulinum toxin A injection after active or passive muscle manipulation. Dev Med Child Neurol 2007; 49(12): 907– 914.
29. Kang BS, Bang MS, Jung SH. Effects of botulinum toxin A therapy with electrical stimulation on spastic calf muscles in children with cerebral palsy. Am J Phys Med Rehabil 2007; 86(11): 901– 906.
30. Cosgrove AP, Corry IS, Graham HK. Botulinum toxin in the management of the lower limb in cerebral palsy. Dev Med Child Neurol 1994; 36(5): 386– 396.
31. Molenaers G, Desloovere K, Fabry G, De Cock P. The effects of quantitative gait assessment and botulinum toxin a on musculoskeletal surgery in children with cerebral palsy. J Bone Joint Surg Am 2006; 88(1): 161– 170.
32. Cosgrove AP, Graham HK. Botulinum toxin A prevents the development of contractures in the hereditary spastic mouse. Dev Med Child Neurol 1994; 36(5): 379– 385.
33. Arnold AS, Blemker SS, Delp SL. Evaluation of a deformable musculoskeletal model for estimating muscle- tendon lengths during crouch gait. Ann Biomed Eng 2001; 29(3): 263– 274.
34. Lukban MB, Rosales RL, Dressler D. Effectiveness of botulinum toxin A for upper and lower limb spasticity in children with cerebral palsy: a summary of evidence. J Neural Transm 2009; 116(3): 319– 331.
35. Kaňovský P, Bareš M, Severa S, Benetin J, Kraus J,Richardson A et al. Functional benefit of botulinum toxin (Dysport) in the treatment of dynamic equinus cerebral palsy spasticity: a prospective, multicentre, double‑blind, placebo- controlled study. Cesk Slov Neurol N 2004; 67/ 100(1): 16– 23.
Štítky
Paediatric neurology Neurosurgery NeurologyČlánok vyšiel v časopise
Czech and Slovak Neurology and Neurosurgery
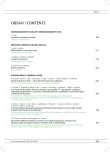
2009 Číslo 6
- Advances in the Treatment of Myasthenia Gravis on the Horizon
- Memantine Eases Daily Life for Patients and Caregivers
- Spasmolytic Effect of Metamizole
Najčítanejšie v tomto čísle
- The Variants of the Catatonia
- Carpal Tunnel Syndrome
- Mental Nerve Neuropathy as a Manifestation of Systemic Malignancy
- Rett Syndrome