Microbiome in connection with metabolic syndrome and the therapeutic potential of its influencing
Authors:
Miriam Tutková; Jana Rudá-Kučerová
Published in the journal:
Čes. slov. Farm., 2018; 67, 71-80
Category:
Review Articles
Summary
Several fields of medicine have been concerned with the role of the microbiome in maintaining the balance in the human body and its changes in the pathogenesis of diseases in recent years. The intestinal microbiome seems to play a key role in the regulation of metabolic pathways, inflammation and intestinal permeability. The aim of this review is to assess the importance of the intestinal microbiome in metabolic syndrome and the therapeutic or preventive potential of its manipulation.
Key words:
metabolic syndrome • microbiome • probiotics • prebiotics • fecal transplant
Zdroje
1. Monda V, et al. Exercise Modifies the Gut Microbiota with Positive Health Effects. Oxid. Med. Cell. Longev. 2017; 2017, 3831972.
2. Cani P. D, et al. Metabolic Endotoxemia Initiates Obesity and Insulin Resistance. Diabetes 2007; 56, 1761–1772.
3. Gibson G. R., Roberfroid M. B. Dietary modulation of the human colonic microbiota: introducing the concept of prebiotics. J. Nutr. 1995; 125, 1401–1412.
4. Backhed F, et al. The gut microbiota as an environmental factor that regulates fat storage. Proc. Natl. Acad. Sci. 2004; 101, 15718–15723.
5. Eckburg P. B, et al. Diversity of the Human Intestinal Microbial Flora. Science 2005; 308, 1635–1638.
6. Zoetendal E. G., Vaughan E. E., De Vos W. M. A microbial world within us. Mol. Microbiol. 2006; 59, 1639–1650.
7. Sun J., Chang E. B. Exploring gut microbes in human health and disease: Pushing the envelope. Genes Dis. 2014; 1, 132–139.
8. Petschow B, et al. Probiotics, prebiotics, and the host microbiome: the science of translation. Ann. N. Y. Acad. Sci. 2013; 1306, 1–17.
9. Ley R. E., Backhed F., Turnbaugh P., Lozupone C. A., Knight R. D., Gordon J. I. Obesity alters gut microbial ecology. Proc. Natl. Acad. Sci. 2005; 102, 11070–11075.
10. Ley R. E., Turnbaugh P. J., Klein S., Gordon J. I. Microbial ecology: Human gut microbes associated with obesity. Nature 2006; 444, 1022–1023.
11. Collado M. C., Isolauri E., Laitinen K., Salminen S. Distinct composition of gut microbiota during pregnancy in overweight and normal-weight women. Am. J. Clin. Nutr. 2008; 88, 894–899.
12. Jumpertz R, et al. Energy-balance studies reveal associations between gut microbes, caloric load, and nutrient absorption in humans. Am. J. Clin. Nutr. 2011; 94, 58–65.
13. Remely M., Hippe B., Zanner J., Aumueller E., Brath H., Haslberger A. G. Gut microbiota of obese, type 2 diabetic individuals is enriched in Faecalibacterium prausnitzii, Akkermansia muciniphila and Peptostreptococcus anaerobius after weight loss. Endocr. Metab. Immune Disord. Drug Targets 2016; 16, 99–106 .
14. Carabotti M., Scirocco A., Maselli M. A., Severi C. The gut-brain axis: interactions between enteric microbiota, central and enteric nervous systems. Ann. Gastroenterol. 2015; 28, 203–209.
15. Brown A. J, et al. The Orphan G protein-coupled receptors GPR41 and GPR43 are activated by propionate and other short chain carboxylic acids. J. Biol. Chem. 2003; 278, 11312–11319.
16. Xiong Y, et al. Short-chain fatty acids stimulate leptin production in adipocytes through the G protein-coupled receptor GPR41. Proc. Natl. Acad. Sci. USA 2004; 101, 1045–1050.
17. Maslowski K. M, et al. Regulation of inflammatory responses by gut microbiota and chemoattractant receptor GPR43. Nature 2009; 461, 1282–1286.
18. de Vadder F, et al. Microbiota-generated metabolites promote metabolic benefits via gut-brain neural circuits. Cell 2014; 156, 84–96.
19. Archer B. J., Johnson S. K., Devereux H. M., Baxter A. L. Effect of fat replacement by inulin or lupin-kernel fibre on sausage patty acceptability, post-meal perceptions of satiety and food intake in men. Br. J. Nutr. 2004; 91, 591.
20. Cani P. D., Dewever C., Delzenne N. M. Inulin-type fructans modulate gastrointestinal peptides involved in appetite regulation (glucagon-like peptide-1 and ghrelin) in rats. Br. J. Nutr. 2004; 92, 521–526.
21. Cani P. D, et al. Changes in gut microbiota control inflammation in obese mice through a mechanism involving GLP-2-driven improvement of gut permeability. Gut 2009; 58, 1091–1103.
22. Roberfroid M, et al. Prebiotic effects: metabolic and health benefits. Br. J. Nutr. 2010; 104, 1–63.
23. Cani P. D., Hoste S., Guiot Y., Delzenne N. M. Dietary non-digestible carbohydrates promote L-cell differentiation in the proximal colon of rats. Br. J. Nutr. 2007; 98, 32–37.
24. Fruhwürth S., Vogel H., Schürmann A., Williams K. J. Novel Insights into How Overnutrition Disrupts the Hypothalamic Actions of Leptin. Front. Endocrinol. (Lausanne). 2018; 9, 89.
25. Everard A, et al. Responses of gut microbiota and glucose and lipid metabolism to prebiotics in genetic obese and diet-induced leptin-resistant mice. Diabetes 2011; 60, 2775–2786.
26. Petersen A. M. W., Pedersen B. K. The anti-inflammatory effect of exercise. J. Appl. Physiol. 2005; 98, 1154–1162.
27. Bäckhed F., Manchester J. K., Semenkovich C. F., Gordon J. I. Mechanisms underlying the resistance to diet-induced obesity in germ-free mice. Proc. Natl. Acad. Sci. 2007; 104, 979–984.
28. Aronsson L, et al. Decreased fat storage by Lactobacillus paracasei is associated with increased levels of angiopoietin-like 4 protein (ANGPTL4). PLoS One 2010; 5, 13087.
29. Rhee S. H., Pothoulakis C., Mayer E. A. Principles and clinical implications of the brain-gut-enteric microbiota axis. Nat. Rev. Gastroenterol. Hepatol. 2009; 6, 306–314.
30. Kabouridis P. S, et al. Microbiota controls the homeostasis of glial cells in the gut lamina propria. Neuron 2015; 85, 289–295.
31. Tennoune N, et al. Bacterial ClpB heat-shock protein, an antigen-mimetic of the anorexigenic peptide α-MSH, at the origin of eating disorders. Transl. Psychiatry 2014; 4, 458.
32. Gusarov I, et al. Bacterial nitric-oxide synthases operate without a dedicated redox partner. J. Biol. Chem. 2008; 283, 13140–13147.
33. Ji X. B.,Hollocher T. C. Reduction of nitrite to nitric oxide by enteric bacteria. Biochem. Biophys. Res. Commun. 1988; 157, 106–108.
34. Williams B. B, et al. Discovery and characterization of gut microbiota decarboxylases that can produce the neurotransmitter tryptamine. Cell Host Microbe 2014; 16, 495–503.
35. Roberts C. K., Hevener A. L., Barnard R. J. Metabolic syndrome and insulin resistance: underlying causes and modification by exercise training. Comprehensive Physiology 2013; 3, 1–58.
36. Horská K., Kučerová J., Suchý P., Kotolová H. Metabolic syndrome – dysregulation of adipose tissue endocrine function. Ces. slov. Farm. 2014; 63, 152–159.
37. Bloch-Damti A, et al. Differential effects of IRS1 phosphorylated on Ser307 or Ser632 in the induction of insulin resistance by oxidative stress. Diabetologia 2006; 49, 2463–2473.
38. Nishimura S, et al. CD8+ effector T cells contribute to macrophage recruitment and adipose tissue inflammation in obesity. Nat. Med. 2009; 15, 914–920.
39. Boutagy N. E., McMillan R. P., Frisard M. I., Hulver M. W. Metabolic endotoxemia with obesity: Is it real and is it relevant? Biochimie 2016; 124, 11–20.
40. Luche E, et al. Metabolic endotoxemia directly increases the proliferation of adipocyte precursors at the onset of metabolic diseases through a CD14-dependent mechanism. Mol. Metab. 2013; 2, 281–291.
41. Thomas C. J, et al. Evidence of a trimolecular complex involving LPS, LPS binding protein and soluble CD14 as an effector of LPS response. FEBS Lett 2002; 531, 184–188.
42. Ghoshal S., Witta J., Zhong J., De Villiers W., Eckhardt E. Chylomicrons promote intestinal absorption of lipopoly-saccharides. J. Lipid Res. 2009; 50, 90–97.
43. Maziere C., Conte M. A., Dantin F., Maziere J. C. Lipopolysaccharide enhances oxidative modification of low density lipoprotein by copper ions, endothelial and smooth muscle cells. Atherosclerosis 1999; 143, 75–80.
44. Kitchens R. L., Thompson P. A., Viriyakosol S., O’Keefe G. E., Munford R. S. Plasma CD14 decreases monocyte responses to LPS by transferring cell-bound LPS to plasma lipoproteins. J. Clin. Invest. 2001; 108, 485–493.
45. Vora P, et al. Beta-defensin-2 expression is regulated by TLR signaling in intestinal epithelial cells. J. Immunol. 2004; 173, 5398–5405.
46. Mantis N. J., Rol N., Corthésy B. Secretory IgA’s complex roles in immunity and mucosal homeostasis in the gut. Mucosal Immunol. 2011; 4, 603–611.
47. Eberl G., Colonna M., Di Santo J. P., McKenzie A. N. J. Innate lymphoid cells: A new paradigm in immunology. Science 2015; 348, 6566.
48. Wang X, et al. Interleukin-22 alleviates metabolic disorders and restores mucosal immunity in diabetes. Nature 2014; 514, 237–241.
49. Dethlefsen L., Huse S., Sogin M. L., D. A. Relman D. A. The pervasive effects of an antibiotic on the human gut microbiota, as revealed by deep 16S rRNA sequencing. PLoS Biol. 2008; 6, 280.
50. Angelakis E., Armougom F., Million M., Raoult D. The relationship between gut microbiota and weight gain in humans. Future Microbiol. 2012; 7, 91–109.
51. Yun S. I., Park H. O., Kang J. H. Effect of Lactobacillus gasseri BNR17 on blood glucose levels and body weight in a mouse model of type 2 diabetes. J. Appl. Microbiol. 2009; 107, 1681–1686.
52. Kang J. H., Yun S. I., Park M. H., Park J. H., Jeong S. Y., Park H. O. Anti-obesity effect of Lactobacillus gasseri BNR17 in high-sucrose diet-induced obese mice. PLoS One 2013; 8, 54617.
53. Kang J. H., Yun S. I., Park H. O. Effects of Lactobacillus gasseri BNR17 on body weight and adipose tissue mass in diet-induced overweight rats. J. Microbiol. 2010; 48, 712–714.
54. Miyoshi M., Ogawa A., Higurashi S., Kadooka Y. Anti-obesity effect of Lactobacillus gasseri SBT2055 accompanied by inhibition of pro-inflammatory gene expression in the visceral adipose tissue in diet-induced obese mice. Eur. J. Nutr. 2014; 53, 599–606.
55. Ji Y. S, et al. Modulation of the murine microbiome with a concomitant anti-obesity effect by Lactobacillus rhamnosus GG and Lactobacillus sakei NR28. Benef. Microbes 2012; 3, 13–22.
56. Yoo S. R, et al. Probiotics L. plantarum and L. curvatus in combination alter hepatic lipid metabolism and suppress diet-induced obesity. Obesity (Silver Spring). 2013; 21, 2571–2578.
57. An H. M, et al. Antiobesity and lipid-lowering effects of Bifidobacterium spp. in high fat diet-induced obese rats. Lipids Health Dis. 2011; 10, 116.
58. Cano P. G., Santacruz A., Trejo F. M., Sanz Y. Bifidobacterium CECT 7765 improves metabolic and immunological alterations associated with obesity in high-fat diet-fed mice. Obesity (Silver Spring) 2013; 21, 2310–2321.
59. Esposito E, et al. Probiotics reduce the inflammatory response induced by a high-fat diet in the liver of young rats. J. Nutr. 2009; 139, 905–911.
60. Aronsson L, et al. Decreased fat storage by Lactobacillus paracasei is associated with increased levels of angiopoietin-like 4 protein (ANGPTL4). PLoS One 2010; 5, 13087.
61. Kim S. W., Park K. Y., Kim B., Kim E., Hyun C. K. Lactobacillus rhamnosus GG improves insulin sensitivity and reduces adiposity in high-fat diet-fed mice through enhancement of adiponectin production. Biochem. Biophys. Res. Commun. 2013; 431, 258–263.
62. Park D. Y, et al. Supplementation of Lactobacillus curvatus HY7601 and Lactobacillus plantarum KY1032 in diet-induced obese mice is associated with gut microbial changes and reduction in obesity. PLoS One 2013; 8, 59470.
63. Kadooka Y, et al. Effect of Lactobacillus gasseri SBT2055 in fermented milk on abdominal adiposity in adults in a randomised controlled trial. Br. J. Nutr. 2013; 110, 1696–1703.
64. Kadooka Y, et al. Regulation of abdominal adiposity by probiotics (Lactobacillus gasseri SBT2055) in adults with obese tendencies in a randomized controlled trial. Eur. J. Clin. Nutr. 2010; 64, 636–643.
65. Gøbel R. J., Larsen N., Jakobsen M., Mølgaard C., Michaelsen K. F. Probiotics to adolescents with obesity: effects on inflammation and metabolic syndrome. J. Pediatr. Gastroenterol. Nutr. 2012; 55, 673–678.
66. Leber B, et al. The influence of probiotic supplementation on gut permeability in patients with metabolic syndrome: an open label, randomized pilot study. Eur. J. Clin. Nutr. 2012; 6610, 1110–1115.
67. Tripolt N. J, et al. Short communication: Effect of supplementation with Lactobacillus casei Shirota on insulin sensitivity, β-cell function, and markers of endothelial function and inflammation in subjects with metabolic syndrome – a pilot study. J. Dairy Sci. 2013;96, 89–95.
68. Jung S. P, et al. Effect of Lactobacillus gasseri BNR17 on Overweight and Obese Adults: A Randomized, Double-Blind Clinical Trial. Korean J. Fam. Med. 2013; 34, 80–89.
69. Woodard G. A, et al. Probiotics improve outcomes after Roux-en-Y gastric bypass surgery: a prospective randomized trial. J. Gastrointest. Surg. 2009; 13, 1198–1204.
70. Bukowska H., Pieczul-Mróz J., Jastrzebska M., Chełstowski K., Naruszewicz M. Decrease in fibrinogen and LDL-cholesterol levels upon supplementation of diet with Lactobacillus plantarum in subjects with moderately elevated cholesterol. Atherosclerosis 1998; 137, 437–438.
71. Agerholm-Larsen L., Raben A., Haulrik N., Hansen A. S., Manders M., Astrup A. Effect of 8 week intake of probiotic milk products on risk factors for cardiovascular diseases. Eur. J. Clin. Nutr. 2000; 54, 288–297.
72. Naruszewicz M., Johansson M. L., Zapolska-Downar D., Bukowska H. Effect of Lactobacillus plantarum 299v on cardiovascular disease risk factors in smokers. Am. J. Clin. Nutr. 2002; 76, 1249–1255.
73. Andreasen A. S., et al. Effects of Lactobacillus acidophilus NCFM on insulin sensitivity and the systemic inflammatory response in human subjects. Br. J. Nutr. 2010; 104, 1831–1838.
74. Ogawa A., Kadooka Y., Kato K., Shirouchi B., Sato M. Lactobacillus gasseri SBT2055 reduces postprandial and fasting serum non-esterified fatty acid levels in Japanese hypertriacylglycerolemic subjects. Lipids Health Dis. 2014; 13, 36.
75. Cani P. D, et al. Selective increases of bifidobacteria in gut microflora improve high-fat-diet-induced diabetes in mice through a mechanism associated with endotoxaemia. Diabetologia 2007; 50, 2374–2383.
76. Everard A, et al. Responses of gut microbiota and glucose and lipid metabolism to prebiotics in genetic obese and diet-induced leptin-resistant mice. Diabetes 2011; 60, 2775–2786.
77. Delzenne N. M., Neyrinck A. M., Cani P. D. Gut microbiota and metabolic disorders: how prebiotic can work? Br. J. Nutr. 2013; 109, 81–85.
78. Dewulf E. M, et al. Inulin-type fructans with prebiotic properties counteract GPR43 overexpression and PPARγ-related adipogenesis in the white adipose tissue of high-fat diet-fed mice. J. Nutr. Biochem. 2011; 22, 712–722.
79. Cani P. D., Dewever C., Delzenne N. M. Inulin-type fructans modulate gastrointestinal peptides involved in appetite regulation (glucagon-like peptide-1 and ghrelin) in rats. Br. J. Nutr. 2004; 92, 521–526.
80. Cani P. D., Joly E., Horsmans Y., Delzenne N. M. Oligofructose promotes satiety in healthy human: a pilot study. Eur. J. Clin. Nutr. 2006; 60, 567–572.
81. Li A. N., Li S., Zhang Y. J., Xu X. R., Chen Y. M., Li H. B. Resources and biological activities of natural polyphenols. Nutrients 2014; 6, 6020–6047.
82. Roopchand D. E, et al. Dietary Polyphenols promote growth of the gut bacterium Akkermansia muciniphila and attenuate high-fat diet – induced metabolic syndrome. Diabetes 2015; 64, 2847–2858.
83. Taira T, et al. Dietary polyphenols increase fecal mucin and immunoglobulin A and ameliorate the disturbance in gut microbiota caused by a high fat diet. J. Clin. Biochem. Nutr. 2015; 57, 212–216.
84. Williamson G., Clifford M. N. Colonic metabolites of berry polyphenols: the missing link to biological activity? Br. J. Nutr. 2010; 104, 48–66.
85. Zhao Y., Li X., Zeng X., Huang S., Hou S., Lai X. Characterization of phenolic constituents in Lithocarpus polystachyus. Anal. Methods 2014; 6, 1359.
86. González-Gallego J., García-Mediavilla M. V., Sánchez-Campos S., Tuñón M. J. Fruit polyphenols, immunity and inflammation. Br. J. Nutr. 2010; 104, 15–27.
87. Kwon O, et al. Inhibition of the intestinal glucose transporter GLUT2 by flavonoids. FASEB J. 2007; 21, 366–377.
88. Hanhineva K, et al. Impact of Dietary Polyphenols on Carbohydrate Metabolism. Int. J. Mol. Sci. 2010; 11, 1365–1402.
89. Stevens J. F., Maier C. S. The chemistry of gut microbial metabolism of polyphenols. Phytochem. Rev. 2016; 15, 425–444.
90. Cardona F., Andrés-Lacueva C., Tulipani S., Tinahones F. J., Queipo-Ortuño M. I. Benefits of polyphenols on gut microbiota and implications in human health. J. Nutr. Biochem. 2013; 24, 1415–1422.
91. Mei X, et al. Insulin sensitivity-enhancing activity of phlorizin is associated with lipopolysaccharide decrease and gut microbiota changes in obese and type 2 diabetes (db/db) Mice. J. Agric. Food Chem. 2016; 64, 7502–7511.
92. Santacruz A, et al. Gut microbiota composition is associated with body weight, weight gain and biochemical parameters in pregnant women. Br. J. Nutr. 2010; 104, 83–92.
93. Masumoto S., Terao A., Yamamoto Y., Mukai T., Miura T., Shoji T. Non-absorbable apple procyanidins prevent obesity associated with gut microbial and metabolomic changes. Sci. Rep. 2016; 6, 31208.
94. Baba S, et al. Bioavailability of (–)-epicatechin upon intake of chocolate and cocoa in human volunteers. Free Radic. Res. 2000; 33, 635–641.
95. Gibson G. R. Dietary modulation of the human gut microflora using the prebiotics oligofructose and inulin. J. Nutr. 1999; 129, 1438–1441.
96. Tzounis X, et al. Flavanol monomer-induced changes to the human faecal microflora. Br. J. Nutr. 2008; 99, 782–792.
97. Hughes R., Magee E. A., Bingham S. Protein degradation in the large intestine: relevance to colorectal cancer. Curr. Issues Intest. Microbiol. 2000; 1, 51–58.
98. Massot-Cladera M., Pérez-Berezo T., Franch A., Castell M., Pérez-Cano F. J. Cocoa modulatory effect on rat faecal microbiota and colonic crosstalk. Arch. Biochem. Biophys. 2012; 527, 105–112.
99. Tzounis X., Rodriguez-Mateos A., Vulevic J., Gibson G. R., Kwik-Uribe C., Spencer J. P. E. Prebiotic evaluation of cocoa-derived flavanols in healthy humans by using a randomized, controlled, double-blind, crossover intervention study. Am. J. Clin. Nutr. 2011; 93, 62–72.
100. Everard A, et al. Cross-talk between Akkermansia muciniphila and intestinal epithelium controls diet-induced obesity. Proc. Natl. Acad. Sci. USA 2013; 110, 9066–9071.
101. Barrett A, et al. Inhibition of α-amylase and glucoamylase by tannins extracted from cocoa, pomegranates, cranberries, and grapes. J. Agric. Food Chem. 2013; 61, 1477–1486.
102. Brandt L. J. Intestinal microbiota and the role of fecal microbiota transplant (FMT) in treatment of C. difficile Infection. Am. J. Gastroenterol. 2013; 108, 177–185.
103. Konturek P. C, et al. Emerging role of fecal microbiota therapy in the treatment of gastrointestinal and extra-gastrointestinal diseases. J. Physiol. Pharmacol. 2015; 66, 483–491.
104. Vrieze A, et al. Transfer of intestinal microbiota from lean donors increases insulin sensitivity in individuals with metabolic syndrome. Gastroenterology 2012; 143, 913–917.
105. Kulecka M, et al. Prolonged transfer of feces from the lean mice modulates gut microbiota in obese mice. Nutr. Metab. (Lond) 2016; 13, 57.
Štítky
Pharmacy Clinical pharmacologyČlánok vyšiel v časopise
Czech and Slovak Pharmacy
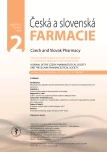
2018 Číslo 2
Najčítanejšie v tomto čísle
- On the content of the National part of the Czech Pharmacopoeia 2017
- Superdisintegrants used in pharmaceutical technology
- Microbiome in connection with metabolic syndrome and the therapeutic potential of its influencing
- Melatonin as a structural template in the development of novel drugs for neurodegenerative disorders