Secondary immunodeficiencies as a result of the iatrogenic impairments
Authors:
Z. Chovancová
Authors‘ workplace:
Ústav klinické imunologie a alergologie, Brno
; Masarykova univerzita, Lékařská fakulta a Fakultní nemocnice u sv. Anny v Brně
Published in:
Reviz. posud. Lék., 23, 2020, č. 1-2, s. 22-31
Category:
Original Articles • Review Articles • Case Reports
Overview
Secondary immunodeficiencies (SID) represent heterogeneous group of acquired impairment of immune systém function with diverse aetiology. It is mostly a combined disorder of both humoral and cellular components of the innate and adaptive immune responses. Except for various pathological states as diabetes mellitus, impairment of liver and kidney functions, protein-energy malnutrition or immunosenescence, also iatrogenic-mediated immune system impairments belong among the most important causes of SID. SID are associated with immunosuppressive or anti-inflammatory treatment used in malignant and autoimmune diseases [(immunosuppressant or disease-modifying drugs, nonsteroidal anti-inflammatory drugs, tyrosine kinase inhibitors or monoclonal antibodies against the CD20 molecule, tumour necrosis factor – (TNF-α) or IL-6 receptor], some other therapeutic procedures (splenectomy or radiotherapy) or present as an adverse events of treatment which at first glance do not interfere with the mechanisms of the immune system (neuroleptic and antiepileptic drugs, angiotensin-converting enzyme inhibitors or anticonvulsants).
Mostly a mild impairment of immunoglobulin production or cellular function is induced, but sometimes clinically significant immunodeficiency develops (especially after treatment with anti-CD20 or anti-TNF-alpha monoclonal antibodies) that already require therapeutic intervention. Therefore, these adverse effects of the treatment should be considered. If patients suffer from clinical symptoms typical for significant immunodeficiency, immunological monitoring is appropriate.
Keywords:
secondary immunodeficiency – radiotherapy – splenectomy – anti-CD20 monoclonal antibodies – anti-TNF-alfa monoclonal antibodies – immunosuppressive drugs – clozapine
Sources
1. Samson, M., Audia, S., Lakomy, D., et al. Diagnostic stratégy for patients with hypogammaglobulinemia in rheumatology. Joint Bone Spine, 2011, 78, p. 241–245.
2. Chinen, J., Shearer, W. T. Secondary immunodeficiencies, including HIV infection. J Allergy Clin Immunol, 2010, 125, p. 195–203.
3. Venhoff, N., Effelsberg, N. M., Salzer, U., et al. Impact of rituximab on immunoglobulin concentrations and B cell numbers after cyclophosphamide treatment in patients with ANCAassociated vasculitides. PLoS One, 2012, 7, e37626.
4. Sistigu, A., Viaud, S., Chaput, N., et al. Immunomodulatory effects of cyclophosphamide and implementations for vaccine design. Semin Immunopathol, 2011, 33, p. 369–383.
5. Ghiringhelli, F., Menard, C., Puig, P. E., et al. Metronomic cyclophosphamide regimen selectively depletes CD4+CD25+ regulátory T cells and restores T and NK effector functions in end stage cancer patients. Cancer Immunol Immunother, 2007, 56, p. 641–648.
6. Sevko, A., Sade-Feldman, M., Kanterman, J., et al. Cyclophosphamide promotes chronic inflammation-dependent immunosuppression and prevents antitumor response in melanoma. J Invest Dermatol, 2013, 133, p. 1610–1619.
7. Becker, J. C., Schrama, D. The dark side of cyclophosphamide: cyclophosphamide-mediated ablation of regulatory T cells. J Invest Dermatol, 2013, 133, p. 1462–1465.
8. Tiede, I., Fritz, G., Strand, S., et al. CD28-dependent Rac1 activation is the molecular target of azathioprine in primary human CD4+ T lymphocytes. J Clin Invest, 2003, 111, p. 1133–1145.
9. Keven, K., Sahin, M., Kutlay, S., et al. Immunoglobulin deficiency in kidney allograft recipients: comparative effects of mycophenolate mofetil and azathioprine. Transpl Infect Dis, 2003, 5, p. 181–186.
10. Bernatsky, S., Hudson, M., Suissa, S. Anti-rheumatic drug use and risk of serious infections in rheumatoid arthritis. Rheumatology (Oxford), 2007, 46, p. 1157–1160.
11. Karnell, J. L., Karnell, F. G., Stephens, G. L., et al. Mycophenolic acid differentially impacts B cell function depending on the stage of differentiation. J Immunol, 2011, 187, p. 3603–3612.
12. Boddana, P., Webb, L. H., Unsworth, J., et al. Hypo-gammaglobulinemia and bronchiectasis in mycophenolate mofetil-treated renal transplant recipients: an emerging clinical phenomenon? Clin Transplant, 2011, 25, p. 417–419.
13. Eickenberg, S., Mickholz, E., Jung, E., et al. Mycophenolic acid counteracts B cell proliferation and plasmablast formation in patients with systemic lupus erythematosus. Arthritis Res Ther, 2012, 14, R110.
14. Goldblum, R. Therapy of rheumatoid arthritis with mycophenolate mofetil. Clin Exp Rheumatol, 1993, 11, p. 117–119.
15. Dhupkar, P., Gordon, N. Interleukin-2: Old and new approaches to enhance immune-therapeutic efficacy. Adv Exp Med Biol, 2017, 995, p. 33–51.
16. Fukata, N., Uchida, K., Kusuda, T., et al. The effective therapy of cyclosporine A with drug delivery system in experimental colitis. J Drug Target, 2011, 19, p. 458–467.
17. Žáčková, D. Tyrozinkinazove inhibitory v lečbě staršich pacientů s chronickou myeloidni leukemii – editorial. Vnitř Lek, 2015, 61, p. 760–761.
18. Santachiara, R., Maffei, R., Martinelli, S., et al. Development of hypogammaglobulinemia in patients treated with imatinib for chronic myeloid leukemia or gastrointestinal stromal tumor. Haematologica, 2008, 93, p. 1252–1255.
19. De Lavallade, H., Khoder, A., Hart, M., et al. Tyrosine kinase inhibitors impair B-cell immune responses in CML through off-target inhibition of kinases important for cell signaling. Blood, 2013, 122, p. 227–238.
20. Kawamata, T., Lu, J., Sato, T., et al. Imatinib mesylate directly impairs class switch recombination through down-regulation of AID: its potential efficacy as an AID suppressor. Blood, 2012, 119, p. 3123–3127.
21. Rajala, H. L. M., Missiry, M. E., Ruusila, A., et al. Tyrosine kinase inhibitor therapy-induced changes in humoral immunity in patients with chronic myeloid leukemia. J Cancer Res Clin Oncol, 2017, 143, p. 1543–1554.
22. Kanis, J. A., Johansson, H., Oden, A., et al. A meta-analysis of prior corticosteroid use and fracture risk. J Bone Miner Res, 2004, 19, p. 893–899.
23. Fauci, A. S., Dale, D. C., Balow, J. E. Glucocorticosteroid therapy: mechanisms of action and clinical considerations. Ann Intern Med, 1976, 84, p. 304–315.
24. Wirsum, C., Glaser, C., Gutenberger, S., et al. Secondary antibody deficiency in glucocorticoid therapy clearly differs from primary antibody deficiency. J Clin Immunol, 2016, 36, p. 406–412.
25. Daien, C. I., Gailhac, S., Mura, T., et al. High levels of memory B cells are associated with response to a first tumor necrosis factor inhibitor in patients with rheumatoid arthritis in a longitudinal prospective study. Arthritis Res Ther, 2014, 16, R95.
26. Hamilos, D. L., Young, R. M., Peter, J. B., et al. Hypogamma-globulinemia in asthmatic patients. Ann Allergy, 1992, 68, p. 472–481.
27. Klaustermeyer, W. B., Gianos, M. E., Kurohara, M. L., et al. IgG subclass deficiency associated with corticosteroids in obstructive lung disease. Chest, 1992, 102, p. 1137–1142.
28. Kawano, T., Matsuse, H., Obase, Y., et al. Hypogamma-globulinemia in steroid-dependent asthmatics correlates with the daily dose of oral prednisolone. Int Arch Allergy Immunol, 2002, 128, p. 240–243.
29. Sharma, S., Lichtenstein, A. Dexamethasone-induced apoptotic mechanisms in myeloma cells investigated by analysis of mutant glucocorticoid receptors. Blood, 2008, 112, p. 1338–1345.
30. Levy, A. L., Waldmann, T. A. The effect of hydrocortisone on immunoglobulin metabolism. J Clin Invest, 1970, 49, p. 1679–1684.
31. Riches, P. G., Hobbs, J. R. Mechanisms in secondary hypogammaglobulinaemia. J Clin Pathol Suppl, 1979, 13, p. 15–22.
32. Butler, W. T., Rossen, R. D. Effects of corticosteroids on immunity in man. I. Decreased serum IgG concentration caused by 3 or 5 days of high doses of methylprednisolone. J Clin Invest, 1973, 52, p. 2629–2640.
33. Galanaud, P., Crevon, M. C., Emilie, D., Abella, A. Effect of hydrocortisone on the in vitro human antibody response: interaction with monocytes and prostaglandins. Clin Immunol Immunopathol, 1983, 29, p. 403–414.
34. Lack, G., Ochs, H. D., Gelfand, E. W. Humoral immunity in steroid-dependent children with asthma and hypogammaglobulinemia. J Pediatr, 1996, 129, p. 898–903.
35. Leandro, M. J. B-cell subpopulations in humans and their dif-ferential susceptibility to depletion with anti-CD20 monoclonal antibodies. Arthritis Res Ther, 2013, 15, S3.
36. Sacco, K. A., Abraham, R. S. Consequences of B-celldepleting therapy: hypogammaglobulinemia and impaired B-cell reconstitution. Immunotherapy, 2018, 10, p. 713–728.
37. McLaughlin, P., Grillo-López, A. J., Link, B. K., et al. Rituximab chimeric anti-CD20 monoclonal antibody therapy for relapsed indolent lymphoma: half of patients respond to a four-dose treatment program. J Clin Oncol, 1998, 16, p. 2825–2833.
38. Lim, S. H., Zhang, Y., Wang, Z., et al. Maintenance rituximab after autologous stem cell transplant for high-risk B-cell lymphoma induces prolonged and severe hypogammaglobulinemia. Bone Marrow Transplant, 2005, 35, p. 207–208.
39. Kosmidis, S., Baka, M., Bouhoutsou, D., et al. Longitudinal assessment of immunological status and rate of immune recovery following treatment in children with ALL. Pediatr Blood Cancer, 2008, 50, p. 528–532.
40. Cabanillas, F., Liboy, I., PaviA, O., RiverA, E. High incidence of non-neutropenic infections induced by rituximab plus fludarabine and associated with hypogammaglobulinemia: a frequently unrecognized and easily treatable complication. Ann Oncol, 2006, 17, p. 1424–1427.
41. Van Vollenhoven, R. F., Fleischmann, R. M., Furst, D. E., et al. Longterm safety of rituximab: final report of the rheumatoid arthritis global clinical trial program over 11 years. J Rheumatol, 2015, 42, p. 1761–1766.
42. Kado, R., Sanders, G., McCune, W. J. Diagnostic and therapeutic considerations in patients with hypogammaglobulinemia after rituximab therapy. Curr Opin Rheumatol, 2017, 29, p. 228–233.
43. Casulo, C., Maragulia, J., Zelenetz, A. D. Incidence of hypogammaglobulinemia in patients receiving rituximab and the use of intravenous immunoglobulin for recurrent infections. Clin Lymphoma Myeloma Leuk, 2013, 13, p. 106–111.
44. Nishio, M., Fujimoto, K., Yamamoto, S., et al. Hypo-gammaglobulinemia with a selective delayed recovery in memory B cells and an impaired isotype expression after rituximab administration as an adjuvant to autologous stem cell transplantation for non-Hodgkin lymphoma. Eur J Haematol, 2006, 77, p. 226–232.
45. De La Torre, I., Leandro, M. J., Edwards, J. C., Cambridge, G. Baseline serum immunoglobulin levels in patients with rheumatoid arthritis: relationships with clinical parameters and with B-cell dynamics following rituximab. Clin Exp Rheumatol, 2012, 30, p. 554–560.
46. Keystone, E., Fleischmann, R., Emery, P., et al. Safety and efficacy of additional courses of rituximab in patients with active rheumatoid arthritis: an open-label extension analysis. Arthritis Rheum, 2007, 56, p. 3896–3908.
47. Králíčková, P., Malá, E., Vokurková, D., et al. Sekundarní humoralní imunodeficience u nemocných se systémovym lupus erythematodes. Vnitř. Lék., 2015, 61, p. 778–794.
48. Kano, G., Nakatani, T., Yagi, K., et al. Complicated pathophysiology behind rituximab-induced persistent hypogammaglobulinemia. Immunol Lett, 2014, 159, p. 76–78.
49. Page, M. J., Bester, J., Pretorius, E. The inflammatory effects of TNF-α and complement component 3 on coagulation. Sci Rep, 2018, 8, p. 1812.
50. Borst, S. E. The role of TNF-alpha in insulin resistance. Endocrine, 2004, 23, p. 177–182.
51. Cawthorn, W. P., Sethi, J. K. TNF-alpha and adipocyte biology. FEBS Lett, 2008, 582, p. 117–131.
52. Tracey, D., Klareskog, L., Sasso, E. H., et al. Tumor necrosis factor antagonist mechanisms of action: a comprehensive review. Pharmacol Ther, 2008, 117, p. 244–279.
53. Mootoo, A., Stylianou, E., Arias, M. A., Reljic, R. TNFalpha in tuberculosis: a cytokine with a split personality. Inflamm Allergy Drug Targets, 2009, 8, p. 53–62.
54. Ten Hove, T., Van Montfrans, C., Peppelenbosch, M. P., Van Deventer, S. J. Infliximab treatment induces apoptosis of lamina propria T lymphocytes in Crohn’s disease. GUT, 2002, 50, p. 206–211.
55. Van Den Brande, J. M., Braat, H., Van Den Brink, G. R., et al. Infliximab but not etanercept induces apoptosis in lamina propria T-lymphocytes from patients with Crohn’s disease. Gastroenterology, 2003, 124, p. 1774–1785.
56. Tak, P. P., Taylor, P. C., Breedveld, F. C., et al. Decrease in cellularity and expression of adhesion molecules by anti-tumor necrosis factor alpha monoclonal antibody treatment in patients with rheumatoid arthritis. Arthritis Rheum, 1996, 39, p. 1077–1081.
57. Pala, O., Diaz, A., Blomberg, B. B., Frasca, D. B lymphocytes in rheumatoid arthritis and the effects of anti-TNF-α agents on B lymphocytes: A review of the literature. Clin Ther, 2018, 40, p. 1034–1045.
58. Moreland, L. W., Bucy, R. P., Weinblatt, M. E., et al. Immune function in patients with rheumatoid arthritis treated with etanercept. Clin Immunol, 2002, 103, p. 13–21.
59. Lee, S. J., Yedla, P., Kavanaugh, A. Secondary immune deficiencies associated with biological therapeutics. Curr Allergy Asthma Rep, 2003, 3, p. 389–395.
60. Mirzaei, A., Mahmoudi, H. Evaluation of TNF-α cytokine production in patients with tuberculosis compared to healthy people. GMS Hyg Infect Control, 2018, 13, Doc09.
61. Seeman, P. Clozapine, a fast-off-D2 antipsychotic. ACS Chem Neurosci, 2014, 5, p. 24–29.
62. Ponsford, M., Castle, D., Tahir, T., et al. Clozapine is associated with secondary antibody deficiency. Br J Psychiatry, 2018, p. 1–7.
63. Lozano, R., Marin, R., Santacruz, M. J., Pascual, A. Selective immunoglobulin M deficiency among clozapine-treated patients: a nested case-control study. Prim Care Companion CNS Disord, 2015, 17.
64. William, B. M., Corazza, G. R. Hyposplenism: a comprehensive review. Part I: basic concepts and causes. Hematology, 2007, 12, p. 1–13.
65. Siebert, A., Gensicka-Kowalewska, M., Cholewinski, G., Dzierzbicka, K. Tuftsin – properties and analogs. Curr Med Chem, 2017, 24, p. 3711–3727.
66. Di Sabatino, A., Carsetti, R., Corazza, G. R. Postsplenectomy and hyposplenic states. Lancet, 2011, 378, p. 86–97.
67. Brigden, M. L. Detection, education and management of the asplenic or hyposplenic patient. Am Fam Physician, 2001, 63, p. 499–506, 508.
68. King, H., Shumacker, H. B. Splenic studies. I. Susceptibility to infection after splenectomy performed in infancy. Ann Surg, 1952, 136, p. 239–242.
69. Price, V. E., Dutta, S., Blanchette, V. S., et al. The prevention and treatment of bacterial infections in children with asplenia or hyposplenia: practice considerations at the Hospital for Sick Children, Toronto. Pediatr Blood Cancer, 2006, 46, p. 597–603.
70. Rubin L. G, Levin M. J, Ljungman P., et al. 2013 IDSA clinical practice guideline for vaccination of the immunocompromised host. Clin Infect Dis, 2014, 58, p. 309–318.
71. Arnott, A., Jones, P., Franklin, L. J., et al. A registry for patients with asplenia/hyposplenism reduces the risk of infections with encapsulated organisms. Clin Infect Dis, 2018.
72. Orth, M., Lauber, K., Niyazi, M., et al. Current concepts in clinical radiation oncology. Radiat Environ Biophys, 2014, 53, p. 1–29.
73. Carvalho, H. A., Villar, R. C. Radiotherapy and immune response: the systemic effects of a local treatment. Clinics (Sao Paulo), 2018, 73, e557s.
74. Venkatesulu, B. P., Mallick, S., LIN, S. H., Krishnan, S. A systematic review of the influence of radiation-induced lymphopenia on survival outcomes in solid tumors. Crit Rev Oncol Hematol, 2018, 123, p. 42–51.
75. Manda, K., Glasow, A., Paape, D., Hildebrandt, G. Effects of ionizing radiation on the immune system with special emphasis on the interaction of dendritic and T cells. Front Oncol, 2012, 2, p. 102.
76. Merrick, A., Errington, F., Milward, K., et al. Immuno-suppressive effects of radiation on human dendritic cells: reduced IL-12 production on activation and impairment of naive T-cell priming. Br J Cancer, 2005, 92, p. 1450–1458.
77. Liu, H., Li, B., Jia, X., et al. Radiation-induced decrease of CD8+ dendritic cells contributes to Th1/Th2 shift. Int Immunopharmacol, 2017, 46, p. 178–185.
78. Formenti, S. C., Demaria, S. Systemic effects of local radiotherapy. Lancet Oncol, 2009, 10, p. 718–726.
79. Compagno, N., Malipiero, G., Cinetto, F., Agostini, C. Immunoglobulin replacement therapy in secondary hypogammaglobulinemia. Front Immunol, 2014, 5, 626.
80. Herriot, R., Sewell, W. A. Antibody deficiency. J Clin Pathol, 2008, 61, p. 994–1000.
Labels
Medical assessment Occupational medicineArticle was published in
Medical Revision
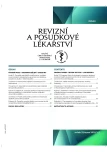
2020 Issue 1-2
Most read in this issue
- Models of health disabled situation and compensation of the consequences
- Secondary immunodeficiencies as a result of the iatrogenic impairments
- Present view of the professional control of health insurance company in conditions of the public insurance system
- The experience of a revision physician from confirming the proposition for spa therapeutic rehabilitation care