FUNCTIONALIZATION OF POLYMERIC NANOFIBERS USING PLATELETS FOR MELANOCYTE CULTURE
Authors:
Karolína Vocetková; Věra Sovková; Matej Buzgo; Radek Divín; Evžen Amler; Eva Filová
Authors place of work:
Institute of Experimental Medicine of the Czech Academy of Sciences, Prague, Czech Republic
Published in the journal:
Lékař a technika - Clinician and Technology No. 1, 2020, 50, 16-22
Category:
doi:
https://doi.org/10.14311/CTJ.2020.1.03
Summary
Tissue engineering is an interdisciplinary field that uses a combination of cells, suitable biomaterials and bioactive molecules to engineer the desired tissue and restore lost function. These principles have quickly begun to spread to the therapy of multiple diseases, including depigmentation disorders. The most common depigmentation disorder is vitiligo, a disease with deep psychosocial implications. Thanks to their unique properties, electrospun polymeric nanofibers represent a material suitable for tissue engineering applications. Furthermore, they may be functionalized with platelets, cells that contain a wide spectrum of growth factors and chemokines. The aim of this paper was to evaluate the functionalization of polymeric nanofibers with platelets and their effects in melanocyte culture. The scaffolds were visualized using scanning electron microscopy, the metabolic activity and proliferation of melanocytes was determined using MTS assay and dsDNA quantification, respectively. Furthermore, the melanocytes were stained and visualized using confocal microscopy. The acquired data showed that poly-ε-caprolactone functionalized with platelets promoted the viability and proliferation of melanocytes. According to the results, such a functionalized scaffold combining nanofibers and platelets may be suitable for melanocyte culture.
Keywords:
Platelets – Tissue engineering – nanofibers
Introduction
Tissue engineering is a dynamically developing medical field aiming to restore, maintain or improve the function of tissues or organs. Its principles were also proposed for the treatment of depigmentation disorders, such as vitiligo. Vitiligo is characterized by acquired, idiopathic and progressive skin and hair depigmentation resulting from loss of functional mela-nocytes [1]. Even nowadays, vitiligo still represents a difficult psychosocial issue, which can lead to de-presssion or anxiety [2, 3]. The concept of a “cellular patch” has been proposed [4], consisting of a suitable biomaterial allowing melanocyte expansion.
Polymeric nanofibers could belong among such suitable biomaterials, as they have been widely used in other tissue engineering applications [5]. One of the most common nanofiber preparation techniques is electrospinning. Electrospinning employs electrical forces to draw submicron fibers from polymer solu-tions and melts. Thanks to their unique properties, electrospun nanofibers have been shown to mimic the structure and biological function of the native extra-cellular matrix (ECM) [6]. These properties include the microarchitecture of the nanofibrous mats, resembling the native ECM, and their high volume-to-surface ratio, facilitating functionalization of nanofibers with bio-active molecules, such as growth factors and cytokines [7].
Platelets; cells involved in blood clotting, represent a promising and versatile source of growth factors. In their alpha granules, they contain supraphysiological levels of a variety of growth factors, chemokines and cytokines. These bioactive molecules include platelet-derived growth factors (PDGF), epidermal growth factor (EGF), transforming growth factor-β (TGF-β), RANTES, monocyte chemoattractant protein-1 (MCP‑1), thrombospondin and fibrinogen [8].
Platelet concentrates are commonly used in trans-fusion medicine as a hemostatic tool. The standard shelf-life of platelet products is 5 days; following that they rapidly lose their hemostatic function [9]. However, it has been shown, that the platelets retain their proliferation potential for up to 21 days [10]. Given the fact that the shelf-life of growth factors is rather short (order of minutes), various drug delivery systems are being developed to prolong their bio-availability and control their spatial-temporal release profile [11].
The aim of this study was to test platelets in combina-tion with nanofibers made of FDA-approved biocom-patible, biodegradable polymers poly-ε-caprolactone (PCL) and polyvinyl alcohol (PVA) to improve the effect of the released bioactive molecules, and to inves-tigate if functionalization with platelets could result in improved melanocyte proliferation.
Methods
Scaffold fabrication & Platelet adhesion
The polycaprolactone (PCL) nanofibers were pre-pared from 24% (w/v) PCL (Sigma-Aldrich, Germany) in a mixture of chloroform and ethanol at a ratio of 9:1, the polyvinyl alcohol (PVA) nanofibers were prepared using 12% (w/v) PVA (Sloviol, Slovakia) with 3% (w/v) glyoxal and 1% phosphoric acid as a crosslinking agent. The samples were fabricated on a Nanospider™ device (Elmarco, Czech Republic) using a wire elec-trode. The distance between the electrode and the collector was 180 mm and the used voltage was 40–60 kV.
Samples with a diameter of 6 mm were punched out of the nanofibers and sterilized using ethylene oxide at 37 °C.
Fresh human leukocyte-poor platelet concentrate derived from buffy-coat (in additive solution) was obtained from Blood Transfusion Service (Šumperk, Czech Republic). The bag was prepared from the blood of four donors. The platelet concentration in the bag was 1,030×109 platelets/L. In total 50 µL of the platelet concentrate was added to the sterile nanofibrous scaf-folds. The platelets were left to adhere to the scaffolds for 2 hours at 22 °C and rinsed with sterile phosphate-buffered saline.
Cell culture & Cell seeding
The melan-a cell line (normal murine pigmented melanocytes) was purchased from the Welcome Trust Functional Genomics Cell Bank (St. George’s, Univer-sity of London). The cells were cultured in DMEM supplemented with 10% fetal bovine serum (FBS), 1% Penicillin/Streptomycin (10,000 IU/mL and 10,000 µg/ml, respectively), 2 mM L-glutamine and 200 nM TPA (12-O-Tetradecanoylphorbol-13-acetate) at 37 °C in 5% CO2. The cell medium was changed every 3 to 4 days. The scaffolds were seeded with me-lan-a cells at a cell seeding density of 12,500 cells/cm2.
Scaffold characterization
The nanofibrous samples were characterized using scanning electron microscopy. Samples containing platelets were rinsed with PBS and fixed with 2.5% glutaraldehyde for four hours at 4 °C. After the fixa-tion, the samples were dehydrated using ethanol changes. Following dehydration, the samples were treated with HMDS (Sigma Aldrich, Germany) and dried. All of the scanned samples were then fixed to a stud and coated with gold (120 s, Quorum Q150RS, 20 mA). Then, the samples were visualized using Vega3 SBU device (Tescan, Czech Republic).
Metabolic activity of melanocytes
The metabolic activity of melanocytes was deter-mined using MTS assay (CellTiter 96® AQueous One Solution Cell Proliferation Assay, Promega, USA). On the experimental days (D1, D3, D6, and D8), 20 µL of the MTS substrate was added to 100 µL of fresh media, and the scaffolds were incubated at 37 °C for 2 hours. Absorbance was detected using an ELISA reader (Infinite M200 Pro, Tecan, Switzerland) at 490 nm. A wavelength of 690 nm was used as a reference wave-length.
Proliferation of melanocytes
Proliferation of melanocytes was determined using a fluorescence kit (Quant-iT™ dsDNA High-Sensi-tivity Assay Kit, Invitrogen, USA). The samples used for the MTS assay were transferred to cell lysis buffer (0.2% Triton X-100, 10 mM Tris, 1 mM EDTA) and were kept at -80 °C until analysis. Then, the samples underwent three freeze-thaw cycles and were pro-cessed according to the manufacturer’s instructions. The fluorescence of the treated samples was read using the ELISA plate reader; excitation wavelength was 485 nm, emission wavelength was 528 nm.
Visualization of cell morphology
The cells were visualized using confocal micros-copy. First, the samples were fixed using frozen metha-nol and stained with DiOC-6 (Life Technologies, USA, CA) to visualize cell biological membranes, and pro-pidium iodide (Sigma-Aldrich, Germany) to visualize cell nuclei. The cell area was determined using Ellipse software (Ellipse, Slovak Republic) from at least 3 independent images. Then, to visualize the viability of the cells, living cells were stained using BCECF-AM (Sigma-Aldrich, Germany) and propidium iodide to visualize dead cells. Viable cells metabolized non-fluorescent BCECF-AM to fluorescent BCECF (green color). Propidium iodide does not penetrate into living cells, therefore only dead cell had red nuclei. The cells were counted using ImageJ software (NIH, USA) from at least 3 independent images, the viability of cells is expressed as percentage of viable cells of the total number of cells present on the scaffolds. The stained samples were observed using LSM 510 DUO confocal microscope (Zeiss, Germany). DiOC-6 (green color; maximum excitation wavelength 484 nm, maximum emission wavelength 501 nm), propidium iodide (red color; maximum excitation wavelength 536 nm, maxi-mum emission wavelength 617 nm), BCECF-AM (green color; maximum excitation wavelength 490 nm, maximum emission wavelength 535 nm).
Statistical analysis
The statistical analysis of the acquired data was performed using the SigmaStat 3.5 software (Systat, USA). The data were tested using ANOVA and Tukey’s test was used for post-hoc analysis. The data are presented as mean ± standard deviation. The level of significance was set to p<0.05.
Results
Nanofibrous scaffold characterization
The fabricated nanofibrous samples were charac-terized using scanning electron microscopy (Fig. 1). The acquired images showed morphology typical for PCL and PVA samples with a minimum of spherical defects.
Throughout the experiment, the presence of platelets on the nanofibrous surface was confirmed by scanning electron microscopy. According to the obtained images, platelets were activated, and a fibrin network was formed on the PCL nanofibers (Fig. 2).
Within the network, activated platelets were detected on day 1. During the experiment, most of the fibrin network was degraded, and on day 8, spherical platelets were still detected on the nanofibers surface. No plate-let adhesion or fibrin network was observed on the surface of PVA nanofibers.
Platelets improve melanocyte adhesion
Adhesion of melanocytes to the nanofibrous scaf-folds was visualized using confocal microscopy 24 hours after cell seeding (Fig. 3). The acquired images were then analyzed using Ellipse software and the cell area was determined.
The acquired images showed melanocytes well spread, with either elongated or polygonal morphology on the PCL nanofibers, in comparison to the rounded or elongated cells detected on the PVA nanofibers. Melanocytes are small cells, therefore the amount of cytoplasm and cell spreading area are relatively small. Furthermore, the dye used for visualization of bio-logical membranes (DiOC-6) exhibited non-specific binding to the PCL nanofibers, visualizing the nano-fibrous network. On the PCL/PL and in some extent on the PVA/PL samples, biological membranes of plate-lets adhered to the nanofibrous samples were detected. The diameter of platelets is about 2–4 µm, which is about 10% of the cell diameter. The data on cell area (Fig. 4) showed that functionalization of PCL nano-fibers with platelets resulted in improved cell spreading and indicated favorable cell culture conditions.
Platelets stimulate melanocyte metabolic activity
To assess the overall biocompatibility of the scaf-folds, the nanofibers were seeded with melanocytes and the metabolic activity of melanocytes was deter-mined using the MTS assay (Fig. 5) on days 1, 3, 6 and 8.
On the PCL scaffolds (both plain and function-alized), the metabolic activity of the seeded melano-cytes was increasing throughout the experiment. The functionalization of PCL scaffolds with platelets (PCL/ PL) resulted in a statistically significant improvement of metabolic activity (p<0.05 on D1; p<0.001 on D3, D6, and D8). There was no significant difference in metabolic activity detected between the PVA and PVA/PL samples. Generally, the metabolic activity of melanocytes seeded on the PVA and PVA/PL samples was very low, suggesting poor cell culture conditions.
Platelets promote proliferation of melanocytes
The proliferation of melanocytes seeded on the nano-fibrous scaffolds was detected on the corresponding samples on days 1, 3, 6, and 8 (Fig. 6). The acquired proliferation data were in concordance with the meta-bolic activity testing. According to Fig. 6, the PVA scaffolds (regardless of platelet functionalization) did not promote proliferation of melanocytes. On the plain PCL scaffold, an increasing rate of proliferation was observed. The functionalization of PCL nanofibers with platelets resulted in a statistically significant in-crease in proliferation of melanocytes on days 3 (p<0.05), 6, and 8 (p<0.001).
Viability visualization of melanocytes
On the last day of the experiment (D8), the melano-cytes seeded on the nanofibrous scaffolds were visual-ized using a vital staining. The detected viable cells are depicted in green, dead cells in red color (Fig. 7).
The scanned images confirmed the data obtained in the proliferation and metabolic activity assays. Further-more, the living and dead cells detected were counted, and the obtained data are summarized in Table 1. The relative high percentage of viable cells on PVA and PVA/PL are caused by the fact that dead cells usually detach from the substrate. However, very low numbers of living cells (green) were observed on both PVA and PVA/PL (Fig. 7).
According to the above-mentioned table, the PCL scaffolds were a suitable scaffold for melanocyte cul-ture. The functionalization of the PCL scaffolds with platelets lead to an almost 2-fold increase in the cell number; the majority of the cells were viable. These data are in accordance with the results of MTS and proliferation testing. PVA scaffolds did not improve cell adhesion and proliferation. Furthermore, more non-viable cells were detected on the PVA scaffolds when compared to the PCL samples.
Discussion
The aim of the study was to test nanofibrous scaf-folds for prospective use for melanocyte expansion. PCL and PVA were chosen as suitable polymers for nanofiber production, as they are biocompatible and biodegradable. PCL has been shown to support adhe-sion and proliferation of multiple cell types [12, 13]. The fabricated nanofibrous scaffolds were examined using scanning electron microscopy and the acquired images showed morphology typical for PCL and PVA samples. Furthermore, the samples contained a mini-mum of spherical defects. The stereology of the scaf-folds was in accordance with other findings [14, 15]. The scanning electron microscope also showed a fine fibrin network formed on the surface of the platelet-functionalized samples. During the experiment, the morphological changes in activated platelets were reversed and spherical platelets were still present on the nanofibers by the end of the experiment. These results are in accordance with Rampichova et al., who ob-served platelets present on centrifugally spun PCL nanofibers after 14 days of the experiment [16]. No platelet adhesion or fibrin network was observed on the surface of PVA nanofibers. This is in accordance with a very low platelet deposition on PVA nanofibers observed by Merkle et al., as PVA lacks cell recog-nition surface sites [17]. The presence of the fibrin network on the PCL nanofibers resulted in improved cell spreading in comparison to the PCL control sam-ple. The influence of the architecture of fibrin fibers on cell spreading was investigated by Bruekers et al., who found that the denser fiber network promoted the spreading of human mesenchymal stem cells [18].
The morphology of the cells was studied on day 1. The total cell area and the amount of cytoplasm de-tected were low and corresponded with typical melano-cyte morphology; elongated or polygonal shape of me-lanocytes was in agreement with our other experiments on PCL nanofibers [19]. It was in contrast to melano-cyte round or irregular elongated morphology on PVA nanofibers which is typical for substrates which do not support cell adhesion.
The confocal images from vital staining do not reflect the cell morphology, but clearly reflect cell viability as only metabolically active cells metabolize the BCECM-AM to green fluorescent dye. However, the evaluation of PVA substrates was influenced by very low cells attached on the substrates which was caused by low proliferation and detachment of dead cells and resulted in relatively high cell viability.
The acquired data are further supported by metabolic activity and proliferation findings. According to the data, PVA nanofibers are not suitable for cell culture regardless of their functionalization with platelets. The cell adhesion properties of PVA nanofibers could be improved using other polymers, such as chitosan [20], gelatin [21] or hyaluronic acid [22]. On the other hand, PCL was shown to be effective in fostering the culture of melanocyte, which is in accordance with previously published data [14, 18]. The suitability of PCL as a substrate for melanocyte culture was described by Savkovic et al. [23]. They were able to differentiate melanocytes from the stem cells of the human hair follicle outer root sheath and cultured them on the PCL nanofibers. The PCL nanofibers were shown to be su-perior to the 2D culture environment in terms of mela-nin production [23]. Functionalization of the nano-fibers with platelets led to further positive stimulation of metabolic activity, viability and proliferation of me-lanocytes when compared to the plain PCL control. The effect may be attributed to the bioactive molecules released from the platelets, as there was no statistically significant difference between the initial number of melanocytes adhered to the PCL and PCL/PL scaffolds on day 1 of the experiment, suggesting the fibrin net-work formed on the surface of the nanofibers improved the spreading of melanocytes but not the absolute numbers of the seeded cells.
Possible limitations of the study include inter-individual platelet variability. The content of bioactive substances may be influenced by several characteristics of the donors, including their age, sex, platelet count and medical conditions [24]. Thus, their effect on cells may be variable in different patients.
Conclusion
This study aimed to test nanofibrous scaffolds that would support the melanocyte culture for prospective use in the treatment of depigmentation disorders. In contrary to PVA nanofibers, the PCL nanofibers with platelets adhered to the surface of nanofibers and represent a promising system. Based on the in vitro data, bioactive molecules released from the platelets significantly influenced melanocyte behavior. Such a facile system delivering a plethora of growth factors and other bioactive molecules while enabling autolo-gous use may be suitable for clinical translation.
Acknowledgement
This work was supported by research grant No. LO1508 of the Ministry of Education, Youth and Sports of the Czech Republic within the National Sustainability Program.
MUDr. Ing. Karolína Vocetková, Ph.D.
Laboratory of Tissue Engineering
Institute of Experimental Medicine
Czech Academy of Sciences
Vídeňská 1083, Praha 4, 142 20
E-mail: karolina.vocetkova@iem.cas.cz
Phone: +420 296 442 387
Zdroje
- Yaghoobi R, Omidian M, Bagherani N. Vitiligo: A review of the published work. Journal of Dermatology. 2011;38:419–31. DOI: 10.1111/j.1346-8138.2010.01139.x
- Alikhan A, Felsten LM, Daly M, Petronic-Rosic V. Vitiligo: A comprehensive overview: Part I. Introduction, epidemiology, quality of life, diagnosis, differential diagnosis, associations, histopathology, etiology, and work-up. Journal of the American Academy of Dermatology. 2011;65:473–91. DOI: 10.1016/j.jaad.2010.11.061
- Talsania N, Lamb B, Bewley A. Vitiligo is more than skin deep: A survey of members of the Vitiligo Society. Clinical and Experimental Dermatology. 2010;35(7):736–9. DOI: 10.1111/j.1365-2230.2009.03765.x
- Lin S-J, Jee S-H, Hsaio W-C, Lee S-J, Young T-H. Formation of melanocyte spheroids on the chitosan-coated surface. Biomaterials. 2005;26:1413–22. DOI: 10.1016/j.biomaterials.2004.05.002
- Pham QP, Sharma U, Mikos AG. Electrospinning of polymeric nanofibers for tissue engineering applications: a review. Tissue Engineering. 2006;12(5):1197–211. DOI: 10.1089/ten.2006.12.1197
- Hassiba AJ, El Zowalaty ME, Nasrallah GK, Webster TJ, Luyt AS, Abdullah AM, et al. Review of recent research on bio-medical applications of electrospun polymer nanofibers for improved wound healing. Nanomedicine. 2016;11(6):715–37. DOI: 10.2217/nnm.15.211
- Ma Z, Kotaki M, Inai R, Ramakrishna S. Potential of Nanofiber Matrix as Tissue-Engineering Scaffolds. Tissue Engineering. 2005;11(1–2):101–9. DOI: 10.1089/ten.2005.11.101
- Burnouf T, Strunk D, Koh MBC, Schallmoser K. Human plate-let lysate: Replacing fetal bovine serum as a gold standard for human cell propagation? Biomaterials. 2016;76:371–87. DOI: 10.1016/j.biomaterials.2015.10.065
- Verma A, Agarwal P. Platelet utilization in the developing world: Strategies to optimize platelet transfusion practices. Transfusion and Apheresis Science. 2009;41(2):145–9. DOI: 10.1016/j.transci.2009.07.005
- Chan RK, Liu P, Lew DH, Ibrahim SI, Srey R, Valeri CR, et al. Expired liquid preserved platelet releasates retain proliferative activity. Journal of Surgical Research. 2005;126(1):55–8. DOI: 10.1016/j.jss.2005.01.013
- Mendes BB, Gómez-Florit M, Babo PS, Domingues RM, Reis RL, Gomes ME. Blood derivatives awaken in regenerative medicine strategies to modulate wound healing. Advanced Drug Delivery Reviews. 2018;129:376–93. DOI: 10.1016/j.addr.2017.12.018
- Shao Z, Zhang X, Pi Y, Wang X, Jia Z, Zhu J, et al. Poly-caprolactone electrospun mesh conjugated with an MSC affinity peptide for MSC homing in vivo. Biomaterials. 2012; 33(12):3375–87. DOI: 10.1016/j.biomaterials.2012.01.033
- Chen M, Patra PK, Warner SB, Bhowmick S. Role of fiber diameter in adhesion and proliferation of NIH 3T3 fibroblast on electrospun polycaprolactone scaffolds. Tissue Engineering. 2007;13(3):579–87. DOI: 10.1089/ten.2006.0205
- Sovkova V, Vocetkova K, Rampichova M, Mickova A, Buzgo M, Lukasova V, et al. Platelet lysate as a serum replacement for skin cell culture on biomimetic PCL nanofibers. Platelets. 2018;29(4). DOI: 10.1080/09537104.2017.1316838
- Buzgo M, Plencner M, Rampichova M, Litvinec A, Prosecka E, Staffa A, et al. Poly-ϵ-caprolactone and polyvinyl alcohol electrospun wound dressings: Adhesion properties and wound management of skin defects in rabbits. Regenerative Medicine. 2019;15(5):423–45. DOI: 10.2217/rme-2018-0072
- Rampichova M, Buzgo M, Mickova A, Vocetkova K, Sovkova V, Lukasova V, et al. Platelet-functionalized three-dimensional poly-ε-caprolactone fibrous scaffold prepared using centrifugal spinning for delivery of growth factors. International Journal of Nanomedicine. 2017;12:347–61. DOI: 10.2147/IJN.S120206
- Merkle VM, Martin D, Hutchinson M, Tran PL, Behrens A, Hossainy S, et al. Hemocompatibility of Polyvinyl Alcohol-Gelatin Core-Shell Electrospun Nanofibers: A Novel Scaffold for Modulating Platelet Deposition and Activation. ACS Applied Materials & Interfaces. 2015;7(15):8302–12. DOI: 10.1021/acsami.5b01671
- Gholipour-Kanani A, Bahrami SH, Joghataie MT, Samadikuchaksaraei A, Ahmadi-Taftie H, Rabbani S, et al. Tissue engineered poly(caprolactone)-chitosan-poly (vinyl alcohol) nanofibrous scaffolds for burn and cutting wound healing. IET Nanobiotechnology. 2014;8(2):123–31. DOI: 10.1049/iet-nbt.2012.0050
- Bruekers SMC, Jaspers M, Hendriks JMA, Kurniawan NA, Koenderink GH, Kouwer PHJ, et al. Fibrin-fiber architecture influences cell spreading and differentiation. Cell Adhesion & Migration. 2016;10(5):495–504. DOI: 10.1080/19336918.2016.1151607
- Yang C, Wu X, Zhao Y, Xu L, Wei S. Nanofibrous scaffold prepared by electrospinning of poly(vinyl alcohol)/gelatin aqueous solutions. Journal of Applied Polymer Sciences. 2011; 121(5):3047–55. DOI: 10.1002/app.33934
- Kim K-O, Akada Y, Kai W, Kim B-S, Kim I-S. Cells Attach-ment Property of PVA Hydrogel Nanofibers Incorporating Hyaluronic Acid for Tissue Engineering. J Biomater Nanobio-technol. 2011;02(04):353–60. DOI: 10.4236/jbnb.2011.24044
- Vocetkova K, Buzgo M, Sovkova V, Bezdekova D, Kneppo P, Amler E. Nanofibrous polycaprolactone scaffolds with adhered platelets stimulate proliferation of skin cells. Cell Proliferation. 2016;49(5). DOI: 10.1111/cpr.12276
- Savkovic V, Flämig F, Schneider M, Sülflow K, Loth T, Lohrenz A, et al. Polycaprolactone fiber meshes provide a 3D environment suitable for cultivation and differentiation of melanocytes from the outer root sheath of hair follicle. Journal of Biomedical Materials Research: Part A. 2016;104(1):26–36. DOI: 10.1002/jbm.a.35832
- Pietramaggiori G, Kaipainen A, Ho D, Orser C, Pebley W, Rudolph A, Orgill DP. Trehalose Lyophilized Platelets for Wound Healing. Wound Repair and Regeneration. 2007; 15(2):213–20. DOI: 10.1111/j.1524-475X.2007.00207.x
Štítky
BiomedicineČlánok vyšiel v časopise
The Clinician and Technology Journal
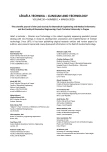
2020 Číslo 1
Najčítanejšie v tomto čísle
- VERIFICATION OF CLINICAL ACCURACY OF AUTOMATED NON-INVASIVE SPHYGMOMANOMETERS: IS IT APPROPRIATE TO USE BLOOD PRESSURE SIMULATORS?
- INFLUENCE OF THE USE OF GRAVITY SETS IN A PRESSURE VOLUMETRIC INFUSION PUMP WITH AN IMPACT ON THE ACCURACY OF INFUSION SOLUTION FLOWS
- FUNCTIONALIZATION OF POLYMERIC NANOFIBERS USING PLATELETS FOR MELANOCYTE CULTURE
- MATERIALS SUITABLE TO SIMULATE SNOW DURING BREATHING EXPERIMENTS FOR AVALANCHE SURVIVAL RESEARCH