The Role of HSF1 Protein in Malignant Transformation
Authors:
Šimončík Oliver; Pastorek Michal; Vojtěšek Bořivoj; Müller Petr
Authors place of work:
Regionální centrum aplikované molekulární onkologie, Masarykův onkologický ústav, Brno
Published in the journal:
Klin Onkol 2018; 31(Supplementum 2): 55-62
Category:
Review
doi:
https://doi.org/10.14735/amko20182S55
Summary
Background:
The heat shock transcription factor, HSF1, is the main regulator of the proteotoxic stress response that orchestrates the adaptation of cells to stress conditions such as elevated temperature, oxidative stress, and proteotoxic stress. As such, HSF1 regulates a large number of stress response-related genes, primarily those encoding heat shock proteins (HSPs). HSPs are molecular chaperones involved in the acquisition of native protein conformations and the prevention of protein degradation, and they also contribute to the removal of denatured proteins via the proteasome. Representative members of the HSP family are HSP70 and HSP90. The stress response is a highly conserved mechanism across all eukaryotes, and HSF1 has been linked to a number of physiological processes (ribosomal biogenesis, translation, transcription, cell cycle, and metabolism) and pathological disorders (neurodegenerative disorders such as Parkinson´s and Alzheimer´s diseases). HSF1 activation is also prominent in different types of cancer (prostate, breast, colorectal carcinoma etc.) where it correlates with tumor aggressiveness and poor prognosis. HSF1 is therefore considered a diagnostic and prognostic marker and is currently being targeted to develop new cancer therapies. Several inhibitors of HSF1 have already been synthesized, but their molecular mechanism (s) of action, specificity those of HSF1, nontoxicity in healthy tissues, and their efficacy in targeting tumor cells remain to be elucidated.
Purpose:
This review summarizes known mechanisms of HSF1 regulation and activation, the role of HSF1 during malignant transformation, and the potential of designing small molecule HSF1 inhibitors for cancer therapy.
Key words:
HSF1 transcription factor – molecular chaperones – cellular stress – tumor transformation – cancer
This work was supported by the project MEYS – NPS I – LO1413.
The authors declare they have no potential conflicts of interest concerning drugs, products, or services used in the study.
The Editorial Board declares that the manuscript met the ICMJE recommendation for biomedical papers.
Accepted: 10. 8. 2018
Zdroje
1. Ritossa F. A new puffing pattern induced by temperature shock and DNP in drosophila. Experientia 1962; 18 (12): 571–573.
2. Tissières A, Mitchell HK, Tracy UM. Protein synthesis in salivary glands of Drosophila melanogaster: relation to chromosome puffs. J Mol Biol 1974; 84 (3): 389–398.
3. Nollen EA, Morimoto RI. Chaperoning signaling pathways: molecular chaperones as stress-sensing “heat shock” proteins. J Cell Sci 2002; 115 (Pt 14): 2809–2816.
4. Nover L, Bharti K, Döring P et al. Arabidopsis and the heat stress transcription factor world: how many heat stress transcription factors do we need? Cell Stress Chaperones 2001; 6 (3): 177–189.
5. Pirkkala L, Nykänen P, Sistonen L. Roles of the heat shock transcription factors in regulation of the heat shock response and beyond. FASEB 2001; 15 (7): 1118–1131.
6. Westerheide SD, Raynes R, Powell C et al. HSF transcription factor family, heat shock response, and protein intrinsic disorder. Curr Protein Pept Sci 2012; 13 (1): 86–103.
7. Akerfelt M, Morimoto RI, Sistonen L. Heat shock factors: integrators of cell stress, development and lifespan. Nat Rev Mol Cell Biol 2010; 11 (8): 545–555. doi: 10.1038/nrm2938.
8. Muchowski PJ, Wacker JL. Modulation of neurodegeneration by molecular chaperones. Nat Rev Neurosci 2005; 6 (1): 11–22. doi: 10.1038/nrn1587.
9. Mendillo ML, Santagata S, Koeva M et al. HSF1 drives a transcriptional program distinct from heat shock to support highly malignant human cancers. Cell 2012; 150 (3): 549–562. doi: 10.1016/j.cell.2012.06.031.
10. Harrison CJ, Bohm AA, Nelson HC. Crystal structure of the DNA binding domain of the heat shock transcription factor. Science 1994; 263 (5144): 224–227.
11. Morimoto RI. Regulation of the heat shock transcriptional response: cross talk between a family of heat shock factors, molecular chaperones, and negative regulators. Genes Dev 1998; 12 (24): 3788–3796.
12. Trinklein ND, Murray JI, Hartman SJ et al. The role of heat shock transcription factor 1 in the genome-wide regulation of the mammalian heat shock response. Mol Biol Cell 2004; 15 (3): 1254–1261. doi: 10.1091/mbc.e03-10-0738.
13. Hentze N, Le Breton L, Wiesner J et al. Molecular mechanism of thermosensory function of human heat shock transcription factor Hsf1. Elife 2016; 5: pii: e11576. doi: 10.7554/eLife.11576.
14. Zou J, Guo Y, Guettouche T et al. Repression of heat shock transcription factor HSF1 activation by HSP90 (HSP90 complex) that forms a stress-sensitive complex with HSF1. Cell 1998; 94 (4): 471–480.
15. Ali A, Bharadwaj S, O’Carroll R et al. HSP90 interacts with and regulates the activity of heat shock factor 1 in Xenopus oocytes. Mol Cell Biol 1998; 18 (9): 4949–4960.
16. Neef DW, Jaeger AM, Gomez-Pastor R et al. A direct regulatory interaction between chaperonin TRiC and stress-responsive transcription factor HSF1. Cell Rep 2014; 9 (3): 955–966. doi: 10.1016/j.celrep.2014.09.056.
17. Ishiwata J, Kasamatsu A, Sakuma K et al. State of heat shock factor 1 expression as a putative diagnostic marker for oral squamous cell carcinoma. Int J Oncol 2012; 40 (1): 47–52. doi: 10.3892/ijo.2011.1178.
18. Hietakangas V, Ahlskog JK, Jakobsson AM et al. Phosphorylation of serine 303 is a prerequisite for the stress-inducible SUMO modification of heat shock factor 1. Mol Cell Biol 2003; 23 (8): 2953–2968.
19. Wang X, Khaleque MA, Zhao MJ et al. Phosphorylation of HSF1 by MAPK-activated protein kinase 2 on serine 121, inhibits transcriptional activity and promotes HSP90 binding. J Biol Chem 2006; 281 (2): 782–791. doi: 10.1074/jbc.M505822200.
20. Chu B, Zhong R, Soncin F et al. Transcriptional activity of heat shock factor 1 at 37 degrees C is repressed through phosphorylation on two distinct serine residues by glycogen synthase kinase 3 and protein kinases Calpha and Czeta. J Biol Chem 1998; 273 (29): 18640–18646.
21. Dai R, Frejtag W, He B et al. c-Jun NH2-terminal kinase targeting and phosphorylation of heat shock factor-1 suppress its transcriptional activity. J Biol Chem 2000; 275 (24): 18210–18218. doi: 10.1074/jbc.M000958200.
22. Kline MP, Morimoto RI. Repression of the heat shock factor 1 transcriptional activation domain is modulated by constitutive phosphorylation. Mol Cell Biol 1997; 17 (4): 2107–2115.
23. Holmberg CI, Hietakangas V, Mikhailov A et al. Phosphorylation of serine 230 promotes inducible transcriptional activity of heat shock factor 1. EMBO J 2001; 20 (14): 3800–3810.doi: 10.1093/emboj/20.14.3800.
24. Murshid A, Chou SD, Prince T et al. Protein kinase a binds and activates heat shock factor 1. PLoS One 2010; 5 (11): e13830. doi: 10.1371/journal.pone.0013830.
25. Chou SD, Prince T, Gong J et al. mTOR is essential for the proteotoxic stress response, HSF1 activation and heat shock protein synthesis. PLoS One 2012; 7 (6): e39679. doi: 10.1371/journal.pone.0039679.
26. Xu YM, Huang DY, Chiu JF et al. Post-translational modification of human heat shock factors and their functions: a recent update by proteomic approach. J Proteome Res 2012; 11 (5): 2625–2634. doi: 10.1021/pr201151a.
27. Core LJ, Lis JT. Transcription regulation through promoter-proximal pausing of RNA polymerase II. Science 2008; 319 (5871): 1791–1792. doi: 10.1126/science.1150843.
28. Ni Z, Schwartz BE, Werner J et al. Coordination of transcription, RNA processing, and surveillance by P-TEFb kinase on heat shock genes. Mol Cell 2004; 13 (1): 55–65.
29. Hoang AT, Huang J, Rudra-Ganguly N et al. A novel association between the human heat shock transcription factor 1 (HSF1) and prostate adenocarcinoma. Am J Pathol 2000; 156 (3): 857–864. doi: 10.1016/S0002-9440 (10) 64954-1.
30. Santagata S, Hu R, Lin NU et al. High levels of nuclear heat-shock factor 1 (HSF1) are associated with poor prognosis in breast cancer. Proc Natl Acad Sci U S A 2011; 108 (45): 18378–18383. doi: 10.1073/pnas.1115031 108.
31. Cen H, Zheng S, Fang YM et al. Induction of HSF1 expression is associated with sporadic colorectal cancer. World J Gastroenterol 2004; 10 (21): 3122–3126.
32. Fang F, Chang R, Yang L. Heat shock factor 1 promotes invasion and metastasis of hepatocellular carcinoma in vitro and in vivo. Cancer 2012; 118 (7): 1782–1794. doi: 10.1002/cncr.26482.
33. Dai C, Whitesell L, Rogers AB et al. Heat shock factor 1 is a powerful multifaceted modifier of carcinogenesis. Cell 2007; 130 (6): 1005–1018. doi: 10.1016/j.cell.2007.07.020.
34. Craig EA. The heat shock response. CRC Crit Rev Biochem 1985; 18 (3): 239–280.
35. Schulz R, Streller F, Scheel AH et al. HER2/ErbB2 activates HSF1 and thereby controls HSP90 clients including MIF in HER2-overexpressing breast cancer. Cell Death Dis 2014; 5: e980. doi: 10.1038/cddis.2013.508.
36. Meng L, Gabai VL, Sherman MY. Heat-shock transcription factor HSF1 has a critical role in human epidermal growth factor receptor-2-induced cellular transformation and tumorigenesis. Oncogene 2010; 29 (37): 5204–5213. doi: 10.1038/onc.2010.277.
37. Xi C, Hu Y, Buckhaults P et al. Heat shock factor Hsf1 cooperates with ErbB2 (Her2/Neu) protein to promote mammary tumorigenesis and metastasis. J Biol Chem 2012; 287 (42): 35646–35657. doi: 10.1074/jbc.M112.377481.
38. Wiech M, Olszewski MB, Tracz-Gaszewska Z et al. Molecular mechanism of mutant p53 stabilization: the role of HSP70 and MDM2. PLoS One 2012; 7 (12): e51426. doi: 10.1371/journal.pone.0051426.
39. Ciocca DR, Calderwood SK. Heat shock proteins in cancer: diagnostic, prognostic, predictive, and treatment implications. Cell Stress Chaperones 2005; 10 (2): 86–103.
40. Jacobs AT, Marnett LJ. HSF1-mediated BAG3 expression attenuates apoptosis in 4-hydroxynonenal-treated colon cancer cells via stabilization of anti-apoptotic Bcl-2 proteins. J Biol Chem 2009; 284 (14): 9176–9183. doi: 10.1074/jbc.M808656200.
41. Wang GL, Jiang BH, Rue EA et al. Hypoxia-inducible factor 1 is a basic-helix-loop-helix-PAS heterodimer regulated by cellular O2 tension. Proc Natl Acad Sci U S A 1995; 92 (12): 5510–5514.
42. Bindra RS, Schaffer PJ, Meng A et al. Alterations in DNA repair gene expression under hypoxia: elucidating the mechanisms of hypoxia-induced genetic instability. Annals of the New York Academy of Sciences 2005; 1059 (1): 184–195. doi: 10.1196/annals.1339.049.
43. Piret J-P, Cosse J-P, Ninane N et al. Hypoxia protects HepG2 cells against etoposide-induced apoptosis via a HIF-1-independent pathway. Exp Cell Res 2006; 312 (15): 2908–2920. doi: 10.1016/j.yexcr.2006.05.018.
44. Um JH, Kang CD, Bae JH et al. Association of DNA-dependent protein kinase with hypoxia inducible factor-1 and its implication in resistance to anticancer drugs in hypoxic tumor cells. Exp Mol Med 2004; 36 (3): 233–242. doi: 10.1038/emm.2004.32.
45. Shen C, Nettleton D, Jiang M et al. Roles of the HIF-1 hypoxia-inducible factor during hypoxia response in Caenorhabditis elegans. J Biol Chem 2005; 280 (21): 20580–20588. doi: 10.1074/jbc.M501894200.
46. Liu G, Roy J, Johnson EA. Identification and function of hypoxia-response genes in Drosophila melanogaster. Physiol Genomics 2006; 25 (1): 134–141. doi: 10.1152/physiolgenomics.00262.2005.
47. Nakano M, Mann DL, Knowlton AA. Blocking the endogenous increase in HSP 72 increases susceptibility to hypoxia and reoxygenation in isolated adult feline cardiocytes. Circulation 1997; 95 (6): 1523–1531.
48. Kabakov AE, Budagova KR, Bryantsev AL et al. Heat shock protein 70 or heat shock protein 27 overexpressed in human endothelial cells during posthypoxic reoxygenation can protect from delayed apoptosis. Cell Stress Chaperones 2003; 8 (4): 335–347.
49. Baird NA, Turnbull DW, Johnson EA. Induction of the heat shock pathway during hypoxia requires regulation of heat shock factor by hypoxia-inducible factor-1. J Biol Chem 2006; 281 (50): 38675–38681. doi: 10.1074/jbc.M608013200.
50. Zhou J, Schmid T, Frank R et al. PI3K/Akt is required for heat shock proteins to protect hypoxia-inducible factor 1alpha from pVHL-independent degradation. J Biol Chem 2004; 279 (14): 13506–13513. doi: 10.1074/jbc.M310164200.
51. Minet E, Mottet D, Michel G et al. Hypoxia-induced activation of HIF-1: role of HIF-1alpha-Hsp90 interaction. FEBS Lett 1999; 460 (2): 251–256.
52. Kubo M, Li T-S, Kurazumi H et al. Heat shock factor 1 contributes to ischemia-induced angiogenesis by regulating the mobilization and recruitment of bone marrow stem/progenitor cells. PLoS One 2012; 7 (5): e37934. doi: 10.1371/journal.pone.0037 934.
53. Slamon DJ, Godolphin W, Jones LA et al. Studies of the HER-2/neu proto-oncogene in human breast and ovarian cancer. Science 1989; 244 (4905): 707–712.
54. Di Fiore PP, Pierce JH, Kraus MH et al. erbB-2 is a potent oncogene when overexpressed in NIH/3T3 cells. Science 1987; 237 (4811): 178–182.
55. Nahta R. Molecular mechanisms of trastuzumab-based treatment in HER2-overexpressing breast cancer. ISRN Oncol 2012; 2012: 428062. doi: 10.5402/2012/428 062.
56. Zhang Y, Chou SD, Murshid A et al. The role of heat shock factors in stress-induced transcription. Methods Mol Biol Clifton NJ 2011; 787: 21–32. doi: 10.1007/978-1-61779-295-3_2.
57. Ma XM, Blenis J. Molecular mechanisms of mTOR-mediated translational control. Nat Rev Mol Cell Biol 2009; 10 (5): 307–318. doi: 10.1038/nrm2672.
58. Wang Y, Xu W, Yan Z et al. Metformin induces autophagy and G0/G1 phase cell cycle arrest in myeloma by targeting the AMPK/mTORC1 and mTORC2 pathways. J Exp Clin Cancer Res 2018; 37 (1): 63. doi: 10.1186/s13046-018-0731-5.
59. De Craene B, Berx G. Regulatory networks defining EMT during cancer initiation and progression. Nat Rev Cancer 2013; 13 (2): 97–110. doi: 10.1038/nrc3447.
60. Hajra KM, Chen DY, Fearon ER. The SLUG zinc-finger protein represses E-cadherin in breast cancer. Cancer Res 2002; 62 (6): 1613–1618.
61. Carpenter RL, Paw I, Dewhirst MW et al. Akt phosphorylates and activates HSF-1 independent of heat shock, leading to Slug overexpression and epithelial-mesenchymal transition (EMT) of HER2-overexpressing breast cancer cells. Oncogene 2015; 34 (5): 546–557. doi: 10.1038/onc.2013.582.
62. Vander Heiden MG, Cantley LC, Thompson CB. Understanding the Warburg effect: the metabolic requirements of cell proliferation. Science 2009; 324 (5930): 1029–1033. doi: 10.1126/science.1160809.
63. Chen Z, Lu W, Garcia-Prieto C et al. The Warburg effect and its cancer therapeutic implications. J Bioenerg Biomembr 2007; 39 (3): 267–274. doi: 10.1007/s10863-007-9086-x.
64. Kim J, Dang CV. Cancer’s molecular sweet tooth and the warburg effect. Cancer Res 2006; 66 (18): 8927–8930. doi: 10.1158/0008-5472.CAN-06-1501.
65. Le A, Cooper CR, Gouw AM et al. Inhibition of lactate dehydrogenase A induces oxidative stress and inhibits tumor progression. Proc Natl Acad Sci U S A 2010; 107 (5): 2037–2042. doi: 10.1073/pnas.0914433107.
66. Hahn JS, Thiele DJ. Activation of the Saccharomyces cerevisiae heat shock transcription factor under glucose starvation conditions by Snf1 protein kinase. J Biol Chem 2004; 279 (7): 5169–5176. doi: 10.1074/jbc.M311005 200.
67. Zhao YH, Zhou M, Liu H et al. Upregulation of lactate dehydrogenase A by ErbB2 through heat shock factor 1 promotes breast cancer cell glycolysis and growth. Oncogene 2009; 28 (42): 3689–3701. doi: 10.1038/onc.2009.229.
68. Hardie DG. AMP-activated protein kinase—an energy sensor that regulates all aspects of cell function. Genes Dev 2011; 25 (18): 1895–1908. doi: 10.1101/gad.17420111.
69. Welch WJ. The role of heat-shock proteins as molecular chaperones. Curr Opin Cell Biol 1991; 3 (6): 1033–1038.
70. Dai S, Tang Z, Cao J et al. Suppression of the HSF1-mediated proteotoxic stress response by the metabolic stress sensor AMPK. EMBO J 2015; 34 (3): 275–293. doi: 10.15252/embj.201489062.
71. Chen K, Qian W, Li J et al. Loss of AMPK activation promotes the invasion and metastasis of pancreatic cancer through an HSF1-dependent pathway. Mol Oncol 2017; 11 (10): 1475–1492. doi: 10.1002/1878-0261.12 116.
72. Foretz M, Guigas B, Bertrand L et al. Metformin: from mechanisms of action to therapies. Cell Metab 2014; 20 (6): 953–966. doi: 10.1016/j.cmet.2014.09.018.
73. Shah SP, Nooka AK, Jaye DL et al. Bortezomib-induced heat shock response protects multiple myeloma cells and is activated by heat shock factor 1 serine 326 phosphorylation. Oncotarget 2016; 7 (37): 59727–59741. doi: 10.18632/oncotarget.10847.
74. Yoon YJ, Kim JA, Shin KD et al. KRIBB11 Inhibits HSP70 synthesis through inhibition of heat shock factor 1 function by impairing the recruitment of positive transcription elongation factor B to the HSP70 promoter. J Biol Chem 2011; 286 (3): 1737–1747. doi: 10.1074/jbc.M110.179440.
75. Salamanca HH, Fuda N, Shi H et al. An RNA aptamer perturbs heat shock transcription factor activity in Drosophila melanogaster. Nucleic Acids Res 2011; 39 (15): 6729–6740. doi: 10.1093/nar/gkr206.
76. Salamanca HH, Antonyak MA, Cerione RA et al. Inhibiting heat shock factor 1 in human cancer cells with a potent RNA aptamer. PLoS one 2014; 9 (5): e96330. doi: 10.1371/journal.pone.0096330.
77. Vilaboa N, Boré A, Martin-Saavedra et al. New inhibitor targeting human transcription factor HSF1: effects on the heat shock response and tumor cell survival. Nucleic Acids Res 2017; 45 (10): 5797–5817. doi: 10.1093/nar/gkx 194.
Štítky
Paediatric clinical oncology Surgery Clinical oncologyČlánok vyšiel v časopise
Clinical Oncology
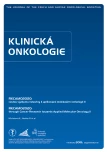
2018 Číslo Supplementum 2
- Metamizole at a Glance and in Practice – Effective Non-Opioid Analgesic for All Ages
- Metamizole vs. Tramadol in Postoperative Analgesia
- Spasmolytic Effect of Metamizole
- Possibilities of Using Metamizole in the Treatment of Acute Primary Headaches
- Current Insights into the Antispasmodic and Analgesic Effects of Metamizole on the Gastrointestinal Tract
Najčítanejšie v tomto čísle
- Effect of DNA Methylation on the Development of Cancer
- Ferroptosis as a New Type of Cell Death and its Role in Cancer Treatment
- Possible Usage of p63 in Bioptic Diagnostics
- Current Methods of microRNA Analysis