Sub-chronic Intra-hippocampal Aminoguanidine Improves Passive Avoidance Task and Expression of Bcl-2 Family Genes in Diabetic Rats
Aminoguanidin podávaný subchronicky intrahipokampálně zlepšuje u diabetických potkanů plnění úkolů pasivního vyhýbání a expresi genů z rodiny Bcl-2
Cíle:
Pozitivní vliv jednotlivých dávek aminoguanidinu (AG) podaných intrahipokampálně na postižení způsobená diabetem u experimentálního zvířecího modelu diabetu již byl popsán. Cílem této studie bylo zjistit účinky 7denního intrahipokampálního podávání injekcí AG na postižení paměti vyvolané diabetem a jeho roli v apoptóze.
Materiály a metodologie:
72 samců potkanů bylo rozděleno do 9 skupin: kontrolní, kontrolní léčená fyziologickým roztokem, kontrolní léčená AG 10, 30 a 90 μg/potkana, diabetici a diabetici léčení AG 10, 30 a 90 μg/potkana. Následně bylo měřeno zvládání pasivního vyhýbání a pomocí RT-PCR geny z rodiny Bcl-2.
Výsledky:
AG významně snížil kognitivní postižení (počet opakování do osvojení si, latence udržení u step-through testu a doba strávená v zatmaveném oddíle) u diabetických potkanů. Léčba AG navíc významně ovlivnila diabetem vyvolané změny v expresi Bax, Bcl-2 a Bcl-xl.
Závěry:
Sedmidenní intrahipokampální injekční aplikace AG může zlepšit zhoršený kognitivní výkon u diabetických potkanů zvýšením Bcl-2 nebo Bcl-xl a snížením poměrů Bax.
Klíčová slova:
diabetes mellitus – aminoguanidin – hipokampus – osvojení pasivního vyhýbání – rodina genů Bcl-2
Autoři deklarují, že v souvislosti s předmětem studie nemají žádné komerční zájmy.
Redakční rada potvrzuje, že rukopis práce splnil ICMJE kritéria pro publikace zasílané do biomedicínských časopisů.
Authors:
M. Alipour 1; A. Aminabadi 1; M. Arab-Firouzjaei 2; B. Amini 1; M. R. Jafari 1
Authors place of work:
Department of Physiology and Pharmacology, School of Medicine, Zanjān University of Medical Sciences, Zanjān, Iran
1; Department of Physiology, School of Medicine, Shiraz University of Medical Sciences, Shiraz, Iran
2
Published in the journal:
Cesk Slov Neurol N 2017; 80/113(5): 584-590
Category:
Original Paper
doi:
https://doi.org/10.14735/amcsnn2017584
Summary
Aims:
The beneficial effect of intra-hippocampal single dose of Aminoguanidine (AG) on deficit in experimental animal model of diabetes has been reported. This study was conducted to investigate the effects of seven-day intra-hippocampal AG injections on memory impairment induced by diabetes mellitus and its role in the apoptosis.
Materials and methodology:
72 male rats were divided into 9 groups: control, control treated with normal saline, control treated with AG 10, 30 and 90 µg/rat, diabetics and diabetics treated with AG 10, 30 and 90 µg/rat. Then the passive avoidance learning and the Bcl-2 family genes was measured by RT-PCR.
Results:
AG significantly ameliorated the cognitive deficits (the number of trials to acquisition, step-through latency of retention and the time spent in the dark compartment) in diabetic rats. Moreover, AG treatment significantly modified the diabetes induced changes in Bax, Bcl–2 and Bcl-xl expression.
Conclusions:
Seven-day intra-hippocampal injections of AG, may improve the impaired cognitive tasks in diabetic rats by increasing either Bcl-2 or Bcl-xl and decreasing Bax ratios.
Key words:
diabetes mellitus – aminoguanidine – hippocampus – passive avoidance learning – Bcl-2 family genes
Chinese summary - 摘要
亚慢性内海马氨基胍改善糖尿病大鼠被动回避任务及Bcl-2家族基因表达目的:
海马内单剂量氨基胍(AG)对糖尿病实验动物模型缺陷的有益效果已被报道。本研究旨在探讨七天海马内注射AG对糖尿病记忆障碍的影响及其在细胞凋亡中的作用。
材料和方法:
将72只雄性大鼠分为以下9组:对照组,生理盐水治疗对照组,分别用10、30和90µg /只剂量AG治疗对照组,糖尿病组,以及分别适用10、30和90µg /只剂量AG治疗糖尿病组。 然后进行被动回避行为学习,并通过RT-PCR测量Bcl-2家族基因。
结果:
AG显着改善了糖尿病大鼠的认知功能障碍(获得试验次数,逐步滞留时间和在暗室中消耗的时间)。 此外,AG治疗显著修复了糖尿病诱导的Bax、Bcl-2和Bcl-xl等基因的表达。
结论:
七天海马内注射AG可能通过增加Bcl-2或Bcl-xl的比率和降低Bax的比率改善糖尿病大鼠的认知障碍。
关键词:
糖尿病 - 氨基胍 - 海马 - 被动回避学习 - Bcl-2家族基因
Introduction
Diabetes mellitus (DM) is a common metabolic disorder characterized by chronic hyperglycemia and disturbances in the metabolism of carbohydrates [1]. Neuropathy is one of the DM complications with nerve regeneration capacity defect being the main factor involved [2,3]. Central complications include impairment of cognitive functions, such as general intelligence, speed of information processing and learning [4]. Pathogenesis of central neuropathy associated with DM is multifactorial [5]. The decrease in brain glutathione levels (antioxidant mechanisms within the cell) and oxidative stress have been suggested as the major factors involved in cognitive deficits [6]. Common mechanisms leading to oxidative stress in DM include either induction of free radical production followed by spontaneous oxidation of glucose or decreased levels of endogenous antioxidant such as A, E, C vitamins and reduction of antioxidant enzyme activities. Therefore, antioxidant treatments may be a suitable therapeutic approach in DM-induced cognitive dysfunctions [7].
Oxidative stress leads to hippocampal neuronal cell death by apoptosis induction [8]. Apoptosis is regulated by two main proteins including caspase enzymes and Bcl-2 family gene products [9]. Bcl-2 family genes include Bcl-2, Bcl-xl, and Bax proteins. Bax product as an apoptotic factor neutralizes the inhibitory effects of both Bcl-2 and Bcl-xl proteins [10]. Aminoguanidine (AG), as an antioxidant, has some biological effects, including inhibition of both amine oxidase and inducible nitric oxide synthase (iNos) and formation of advanced glycation end products (AGEs) [11].
Experimental models demonstrate the protective effect of AG, such as peripheral nerves functional and structural abnormalities induced by DM [12], stroke [13] and transient focal cerebral ischemia [14]. The effects of AG on memory of animals have shown controversial, both positive [15–19] and negative [20–23], results. However, there are a few studies on the effect of AG on memory and anti-apoptotic genes in diabetic animals. We have recently showed that the single intra-hippocampal injection of AG (30 µg/rat) improved memory retrieval in step-through passive avoidance task in diabetic rats, associated with reduced apoptotic and increased anti-apoptotic gene expressions [24]. Also, one week intraperitoneally AG (100 and 200 mg/kg) improved diabetes-associated cognition insufficiency in diabetic rats by enhancing (Bcl-2 + Bcl-xl)/(Bak + Bax) proportions [25]. However, there is no study regarding sub-chronic or chronic effects of intra-hippocampal administration of AG on memory and anti-apoptotic gene expressions in diabetic animals. Our hypothesis was that repeated intra-hippocampal AG injection (10, 30 and 90 µg/rat) could also improve memory deficit induced by DM.
The present study was conducted to investigate the effect of seven-day intra-hippocampal AG injections (10, 30 and 90 µg/rat) on step-through passive avoidance memory impairment induced by DM as well as its role in apoptosis by measuring expression of Bcl-2 family genes.
Material and Methodology
Animals
The animal maintenance and procedures were carried out according to the Principles of Laboratory Animal Care (NIH publication no. 86– 23 revised 1985) and were accepted by the Institutional Animal Ethical Committee (IAEC no. A-10-141-4). Healthy adult male Wistar rats weighing 232–274 g (7–9 weeks) were randomly selected and housed under controlled conditions in a 12 h light/ dark cycle. The rats were fed with a standard laboratory diet and clean drinking water ad libitum.
Surgical procedures
The surgical processes were performed under ketamine-xylazine (100–25 mg/kg) anesthesia with animals being subsequently fixed with a stereotaxic device. A mid-line incision was made along the top of the head, to expose bregma; a stainless steel, 22-gauge guide cannula was inserted (bilaterally) 1 mm above the planned injection site. Stereotactic coordinates for the CA1 regions of the dorsal hippocampi were: anterior (AP) 3–3.5 mm, lateral (LAT) ± 1.8–2 mm and ventral (DV) 2.8–3 mm from the bregma, the cannula was fixed to the skull with dental acrylic [24]. Dummy guides were set into the cannula to avoid blockage by debris to enter the brain. When the experimental sessions had been finished, animals were killed with an overdose of chloroform before bilateral intra-CA1 injection of ink (1% aquatic methylene blue solution). Subsequently, the brain was extracted and fixed with 10% formalin solution for 10 days before sectioning. Sections were examined to control the location of the cannula aimed for the CA1.
Diabetes induction
Diabetes was produced by a single intra-peritoneal (i.p.) injection of 50 mg/kg of streptozotocin [26]. Fasting blood glucose levels were measured 72 hours after the STZ injection. Animals were considered diabetic if their plasma glucose levels were between 13.89 to 22.22 mmol/dl and all groups were homogenous in glycemia.
Animal groups
Following surgery, the animals were allowed recovery for at least one week. The animals were divided into nine equal groups as shown in Tab. 1 (n = 8 rats per groups).
Drug treatments
When diabetes was established, they received intra-hippocampal injection of the saline, AG10, 30 or 90 µg/ rat daily for 7 days, for sub-chronic evaluation of AG treatment on memory retrieval and Bcl-2 family genes in long-term STZ-induced diabetic rat outcomes. For drug administration, the animals were gently restrained by hand; the dummy guides were removed from the guide-cannula and replaced with 27-gauge injection needles (1 mm lower than the tip of guide-cannula). Each injection unit was joined by polyethylene tubing to 1 µl Hamilton syringe. The left and right CA1 regions were infused with a 0.5 µl solution on each side (1 µl/ rat) over a 60-sec period. The injection needles were left in place for additional 60 sec to permit diffusion, then the dummy guides were reinserted into the guide cannula [27]. Body weights were evaluated weekly, diabetic status was reconfirmed after 7 weeks after which learning and memory were also evaluated.
Passive avoidance learning (PAL) step through test
Behavioral studies were evaluated by the shuttle box apparatus. The apparatus and procedure were essentially the same as described in prior studies [28]. Briefly, the step-through passive avoidance apparatus consisted of a lighted chamber (20 × 20 × 30 cm) made of transparent plastic and a dark chamber with dark opaque plastic walls (20 × 20 × 30 cm). The floor of both chambers was made of stainless steel rods (3 mm diameter) spaced 1 cm apart. The floor of the dark chamber could be electrified using a shock generator. A rectangular opening (6 × 8 cm) was placed between the two chambers and could be closed by an opaque guillotine door.
Training
Experimental groups received two trials to habituate them to the apparatus initially. For these trials, the rats were placed in light compartment of the apparatus facing away from the door and 10 sec later the guillotine door was upraised. The rats have natural preference for the dark environment. Once the rat entered the dark compartment, the door was closed and after 30 sec the rats were taken out from the dark compartment and placed in their home cage. The habituation trial was repeated after 30 min and followed after the same interval by the first acquisition trial. The entrance latency to the dark compartment (step-through latency of acquisition, STLa) was recorded when the animal had placed all four paws in the dark compartment.
For the training of the animals, once they had spontaneously entered the dark compartment, the guillotine door was lowered and a mild electrical shock (0.5 mA) was applied for 3 sec, after 30 sec, the rat was returned to its home cage. Then after 2 min, the procedure was repeated. The rat received a foot-shock each time it reentered the dark compartment and had placed all its four paws in the dark compartment but training was terminated when the rat remained in the light compartment for 120 consecutive sec. The number of trials (entries into the dark chamber, TA) was recorded.
Retention test
Long-term memory retrieval was evaluated 24 hours after the PAL acquisition trial. The rats were placed in the lighted chamber as in PAL training session and 10 sec later, the guillotine door was elevated, and the step-through latency (STLr) and the time spent in the dark compartment (TDC) were recorded for up to 600 sec. If the rat did not enter the dark compartment within 600 sec, the retention test was terminated and an upper limit of 600 sec was assigned. During this session, the electric shocks were not applied to the grid floor [29].
At the end of the experiments, weight and blood glucose level of the rats were measured. Formerly, animals were anesthetized with chloroform and the skull was opened along the midline and the brain was removed and placed on an ice-cooled cuttingboard. The meninges were carefully removed and hippocampus was dissected from hemispheres, snapped frozen in liquid nitrogen and stored at –70 °C for extraction of RNA.
RNA Preparation and Semi-quantitative Reverse Transcription Polymerase Chain Reaction (RT-PCR)
Total RNA was extracted from hippocampi using Trizol Reagent (invitrogen), according to the manufacturer’s instructions. Reverse transcription (RT) was performed using 1000 ng of total RNA into cDNA with Revert Aid (Thermo, Scientific RevertAide cDNA synthesis Kit) according to the manufacturer’s instructions, and cDNA samples were stored at – 70 °C. A semi-quantitative reverse transcriptase polymerase chain reaction (RT-PCR) was carried out to determine the levels of Bcl-2, Bcl-xL, and Bax mRNA expressions. The RT-PCR mixture for Bcl-2, Bcl-xL and Bax genes (final volume of 25 µl) contained 2 µl of cDNA, 12.5 µl of Thermo Scientific PCR Master Mix 2× (Qiagen, Germany) and 10 pmols of each complementary primer specific for Bcl-2, Bcl-xL, and Bax sequences as well as for GAPDH (Glyceraldehydes-3-phosphate dehydrogenase; GAPDH gene) sequence as an internal control (Tab. 2). Primers for PCR were designed by means of published cDNA sequences and analyzed with BLASTA program. The samples were denatured at 95 °C for 15 min, and amplified using 40 cycles of 95 °C for 30 sec, 60 °C for 80 sec, and 72 °C for 45 sec for Bax gene and 5 cycles of 95 °C for 30 sec, 62 °C for 80 sec, and 72 °C for 30 sec, as well as 25 cycles of 95 °C for 30 sec, 64 °C for 80 sec and 72 °C for 30 sec for Bcl-2 and Bcl-xL genes followed by a final elongation at 72 °C for 3 min on a Corbett Research thermocycler (Sydney, Australia). The best numbers of cycles were selected for amplification of all genes experimentally so that amplifications were in the exponential ranges and did not reach a plateau. All RT-PCR and PCR reactions contained the use of water in place of a template as a negative control. Eight µl of the final amplification product were run on a 2% ethidium-stained agarose gel and photographed. Finally, the densities of the bands on the agarose gels were determined by the NIH image program. Data were normalized according to the density of the GAPDH bands [24].
Statistical analyses
Data were analyzed using either two ways ANOVA, then Tukeys’ posthoc statistics for parametric or Kruskall-Wallis tests followed by Mann Whitney’s U test for non-parametric data. After Mann Whitney U test, the Holms Bonferoni’s correction was used. Probability values of less than 0.05 were considered significant.
Results
Behavioral results – PAL step through test
Effects of AG treatment on STLa: There were no significant differences in the STLa, demonstrating a lack of sensory and motor disorders in animals. Two ways ANOVA’s F (8.42) = 1.62, P = 0.137 (Fig. 1A).
Effects of AG treatment on the TA: Two-way ANOVA of the mean ± SE of the TAs, showed that 7-day intra-hippocampal injections of AG has an affirmative effecton diabetes-induced impaired TA (F (8.42) = 3.59, P = 0.001, Tukey’s posthoc, P = 0. 002 and 0.001 for 10 and 30 µg/ rats AG, resp., in comparison to diabetic control animals) (Fig. 1B).
Effects of AG treatment on STLr: Based on non-parametric Kruskall-Wallis test of median ± quartile of the step-through latency times, there were no significant differences in the STLr in non-diabetic animals (left columns of the Fig. 1), indicating that neither surgery nor AG alone showed any effect on STLr (H (4) = 4.55, P = 0.34). On the other hand, non-parametric Kruskall-Wallis test showed that 7-day intra-hippocampal injections of AG had significant positive effect on diabetes-induced impaired STLr (7 weeks after induction of diabetes, right columns) (H (3) = 11.05, P = 0.026, Mann Whitney’s U test, P = 0.006, 0.658 and 0.798 for 10, 30 and 90 µg/rats AG resp.). As a result, the 10 µg of the drug / rat could reverse the impaired latency of retention induced by diabetes (Fig. 1C).
Effects of AG treatment on the time spent in TDC: Based on two-way ANOVA of mean ± SE of the TDCs, 7-day intra-hippocampal injections of AG showed a positive effect on diabetes-induced impaired TDC within 10 min [factor A × B, F (8.42) = 7.51, P < 0.0001, Tukey’s posthoc, P = 0.00002, 0.00003 and 0.001 for 10, 30 and 90 µg/ rats AG resp. in comparison to diabetic control animals] (Fig. 1D).
Semi-quantitative mRNA levels of genes regulating cell death: Two-way ANOVA of the mean ± SE, showed that 7-day intra-hippocampal injections of AG has a positive effect on diabetes-induced decrease of both Bcl-2 (F (8.42) = 12.05, P < 0.001, Tukey’s posthoc, P < 0.0001 for 10, 30 and 90 µg/ rts AG resp. in comparison to diabetic control animals) (Fig. 3A) and Bcl-xl expressions (F (8.42) = 41.93, P < 0.001, Tukey’s posthoc, P < 0.0001 for 10, 30 and 90 g/ rats AG resp. in comparison to diabetic control animals). As shown in Fig. 3B, 90 µg/ rats AG had dual effects, it decreased the expression of Bcl-xl genes in non-diabetic but had an opposite effect in the diabetic group.
On the other hand, 7-day intra-hippo-campal injections of AG effectively diminished the diabetes-induced increase of Bax expression (F (8.42) = 17.19, P < 0.001, Tukey’s posthoc, P < 0.0001, P = 0.001 and 0.003 for 10, 30 and 90 µg/ rats AG, resp., in comparison to diabetic control animals) (Fig. 3C).
Discussion
Our results showed that seven weeks after diabetes induction, STLr, TDC and TA impairment was restored by 7-day intra-hippocampal injections of AG. There are several studies indicating the effects of AG on memory. It has been shown that neuronal death and spatial memory impairments induced by lipopolysaccharides were prevented by AG [30]. Memory recovering effect of naringin in unstressed and stressed mice was improved by AG administration significantly as well [16]. Moreover, AG reverted considerably the impairment of learning and memory induced by arsenic [17]. Treatment with AG also antagonized retrograde memory impairment due to hypoxia [18]. Learning and memory deficits caused by aluminum chloride might be prevented by AG treatment [19]. On the other hand, the improving effect of atorvastatin on short-spatial recognition memory was reversed by AG [20]. In morphine-induced memory impairment, improving effect of pioglitazone in the passive avoidance task has not been modified but has reversed in Y-maze discrimination by AG [22]. Moreover, the positive effects of both atorvastatin and granisetron on memory consolidation in scopolamine-treated mice have been significantly reversed by AG [21,23].
In the present study, locomotor activity of the animals was not measured but the effect of AG on STLr can be attributed to unimpaired sensory or locomotor function since the delay in the acquisition phase (STLa) (before electrical shock) showed no significant differences between controls and the experimental group. There is little evidence regarding the effect of AG on memory deficit induced by diabetes in animals. In this study, the best STLr result was obtained with 10 µg/rat AG after 7 days of intra-hippocampal injections of the drug. This is in agreement with the results obtained with a higher single dose of AG (30 µg/ rat) in our previous study [24].
On the other hand, repeated AG administration (10,30 and 90 µg/ rat) indicated significant positive effects on the other parameters (TDC and TA), also similar to our previous study (single dose intrahippocampal injection of the drug) [24].
Several factors are involved in the pathogenesis of diabetes-induced learning and memory deficits [5]. The beneficial effect of AG may be related to its restorative role on a variety of pathogenic processes [31]. It has been shown that AG can improve vascular and neuronal complications detected in experimentally-induced diabetes [32]. Moreover, AGEs play a principal role in learning and memory impairments induced by diabetes, AG can prevent the formation of AGEs [33– 36]. Also, reactive oxygen species (ROS) and reactive nitrogen species (RNS)-induced oxidative damage are other main causes of cognitive dysfunction in diabetes [37] related to iNOS production [38]. Inhibition of iNOS by AG may be related to amelioration of diabetes-induced cognitive dysfunction [39]. Another mechanism of AG-induced neuroprotection may be attributed to its free radical scavenging properties [36, 40]. In addition, AG neuroprotective effects can be featured to a variety of other cellular metabolic pathways [41], including formation of methylglyoxal that acts as an endogenous toxic compound [42] as well as a potent source of ROS [43]. These are frequently accumulated in the hippocampal neurons under conditions of hyperglycemia and impaired glucose metabolism [44,45] and their production can be inhibited by AG [46].
In the present study, diabetes caused decrease in Bcl-2 and Bcl-xl (as anti-apoptotic genes) and increase in Bax (as an apoptotic gene) expression. Consistent with our study, it has been shown that increased Bax expression and caspase-3 activity in a diabetic hippocampus can cause neuronal cell death [47]. Hippocampal neuron apoptosis has an essential role in the learning and memory impairment in diabetes [48,49]. Furthermore, it has been revealed that either reactive methylglyoxal [50,51] or RNS [44,45] are the components inducing apoptosis in the hippocampus by altering expression of Bcl-2 family proteins in the neurons. The pro- and anti-apoptotic balance signals of the Bcl-2 family have a critical role in the release of apoptogenic mitochondrial mediators [52,53]. It has been demonstrated that the mitochondrial pathway plays a major role in apoptosis due to diabetes in hippocampus of STZ-induced diabetic rats [54,55].
Our results demonstrated that 7-day intra-hippocampal injections of AG had a positive effect on diabetes-induced decrease of both Bcl-2 and Bcl-xl expressions. On the other hand, the drug significantly diminished the diabetes-induced increase of Bax expression.
To our knowledge, studies regarding the effect of AG on the mentioned gene expressions in diabetic animal’s hippocampus are lacking. It has been shown that AG as an antioxidant and amine oxidase and iNos inhibitor can inhibit apoptotic cell death [50]. Therefore, the benefitial effects of AG on memory might be related to alteration of apoptosis in the brain areas involved in learning and memory. It is clear that the ratio of Bcl-2 or Bcl-xl to Bax expression determines cell fate and cell leading to the death or life [13]. It has been reported that hyperglycemia induced by STZ results in apoptosis of cortical neurons of newborn rats by increasing the Bax/Bcl-2 ratio [56]. The positive effect of repeated AG administration (10, 30 and 90 µg/rat) on Bax, Bcl-2 and Bcl-xl expression in diabetic animals are in agreement with our previous studies (single dose intra-hippocampal (30 µg/rat) and intraperitoneal (100 and 200 mg/kg) injection of the drug) [24,25].
Much more reliable results could be obtained by real-time PCR and protein level measurements and this is considered as a limitation of our study.
In conclusion, 7-day intra-hippocampal injections of AG may improve impaired cognitive tasks in diabetic rats by increasing either Bcl-2 or Bcl-xl and decreasing Bax ratios. However, additional pathological and molecular investigations are required to explain the detailed mechanisms underlying the neuroprotective effect of AG on memory in diabetic rats.
This study was supported by a research grant from Zanjān University of Medical Sciences, Zanjān, Iran.
The authors declare they have no potential conflicts of interest concerning drugs, products, or services used in the study.
The Editorial Board declares that the manuscript met the ICMJE “uniform requirements” for biomedical papers.
Dr. Mohammad-Reza Jafari
Department of Physiology and Pharmacology
School of Medicine, Zanjān University of Medical Sciences
Gavazang Road, Zanjān
Iran
e-mail: jafarimrj@yahoo.com
Accepted for review: 2. 5. 2017
Accepted for print: 27. 7. 2017
Zdroje
1. Zhang Y, Ren C, Lu G, et al. Anti-diabetic effect of mulberry leaf polysaccharide by inhibiting pancreatic islet cell apoptosis and ameliorating insulin secretory capacity in diabetic rats. Int Immunopharmacol 2014;22(1):248– 57. doi: S1567-5769(14)00253-7.
2. Yasuda H, Terada M, Maeda K, et al. Diabetic neuropathy and nerve regeneration. Prog Neurobiol 2003;69(4):229– 85.
3. Edwards J, Vincent A, Cheng H, et al. Diabetic neuropathy: mechanisms to management. PharmacolTher 2008;120(1):1– 34.
4. Northam E, Anderson P, Jacobs R, et al. Neuropsychological profiles of children with type 1 diabetes 6 years after disease onset. Diabetes Care 2001;24(9):1541– 6.
5. Patil C, Singh V, Kulkarni S. Modulatory effect of sildenafil in diabetes and electroconvulsive shock-induced cognitive dysfunction in rats. Pharmacological Reports 2006;58(3):373– 80.
6. Fukui K, Omoi N, Hayasaka T, et al. Cognitive impairment of rats caused by oxidative stress and aging, and its prevention by vitamin E. Ann N Y Acad Sci 2002;959: 275– 84.
7. Hawkins C,Davies M. Generation and propagation of radical reactions on proteins. Biochim Biophys Acta 2001;1504(2– 3):196– 219.
8. Li ZG, Zhang W, Sima AA. C-peptide prevents hippo-campal apoptosis in type 1 diabetes. Int J Exp Diabetes Res 2002;3(4):241– 5.
9. Thornberry NA, Lazebnik Y. Caspases: enemies within. Science 1998;281(5381):1312– 6.
10. Kroemer G. The proto-oncogene Bcl-2 and its role in regulating apoptosis. Nat Med 1997;3(6):614– 20.
11. Chan A, Cheung M, Law S, et al. Phase II study of alpha-tocopherol in improving the cognitive function of patients with temporal lobe radionecrosis. Cancer 2004;100(2):398– 404.
12. Yagihashi S, Kamijo M, Baba M, et al. Effect of aminoguanidine on functional and structural abnormalities in peripheral nerve of STZ-induced diabetic rats. Diabetes 1992;41(1):47– 52.
13. Sun M, Zhao Y, Gu Y, et al. Neuroprotective actions of aminoguanidine involve reduced the activation of calpain and caspase-3 in a rat model of stroke. Neurochem Int 2010;56(4):634– 41. doi: 10.1016/ j.neuint.2010.01.009.
14. Vakili A, Zahedi-Khorasani M. Effect of aminoguanidine on post-ischemic damage in rodent model of stroke. Pak J Pharm Sci 2008;21(1):24– 8.
15. Liu H, Chen JP, Zhang WQ. [Inducible nitric oxide synthase induces beta-amyloid neurotoxicity in vivo]. Zhongguo Ying Yong Sheng Li Xue Za Zhi 2002;18(4):329– 32.
16. Maratha SR, Mahadevan N. Memory enhancing activity of naringin in unstressed and stressed mice: possible cholinergic and nitriergic modulation. Neurochem Res 2012;37(10):2206– 12. doi: 10.1007/ s11064-012-0844-8.
17. Sharma B, Sharma PM. Arsenic toxicity induced endothelial dysfunction and dementia: pharmacological interdiction by histone deacetylase and inducible nitric oxide synthase inhibitors. Toxicol Appl Pharmacol 2013;273(1):180– 8. doi: 10.1016/ j.taap.2013.07.017.
18. Udayabanu M, Kumaran D, Nair RU, et al. Nitric oxide associated with iNOS expression inhibits acetylcholinesterase activity and induces memory impairment during acute hypobaric hypoxia. Brain Res 2008;1230:138– 49.
19. Stevanovic ID, Jovanovic MD, Colic M, et al. Nitric oxide synthase inhibitors protect cholinergic neurons against AlCl3 excitotoxicity in the rat brain. Brain Res Bull 2010;81(6):641– 6. doi: S0361-9230(10)00006-7.
20. Javadi-Paydar M, Rayatnia F, Fakhraei N, et al.Atorvastatin improved scopolamine-induced impairment in memory acquisition in mice: involvement of nitric oxide. Brain Res 2011;1386:89– 99.
21. Rayatnia F, Javadi-Paydar M, Allami N, et al. Nitric oxide involvement in consolidation, but not retrieval phase of cognitive performance enhanced by atorvastatin in mice. Eur J Pharmacol 2011;666:1– 3):122– 30. doi: S0014-2999(11)00547-4.
22. Babaei R, Javadi-Paydar M, Sharifian M, et al. Involvement of nitric oxide in pioglitazone memory improvement in morphine-induced memory impaired mice. Pharmacol Biochem Behav 2012;103(2):313-21. doi: S0091-3057(12)00239-0.
23. Javadi-Paydar M, Zakeri M, Norouzi A, et al. Involvement of nitric oxide in granisetron improving effect on scopolamine-induced memory impairment in mice. Brain Res 2012;1429:61– 71. doi: S0006-8993(11)01442-9.
24. Arab Firouzjaei M, Jafari MR, Eskandari M, et al. Aminoguanidine Changes Hippocampal Expression of Apoptosis-Related Genes, Improves Passive Avoid-ance Learning and Memory in Streptozotocin-InducedDiabetic Rats. Cellular and Molecular Neurobiology 2014; 34(3):343– 50.
25. Alipour M, Amini B, Adineh F, et al. Effect of sub-chronic intraperitoneal administration of aminoguanidine on the memory and hippocampal apoptosis-related genes in diabetic rats. Bratisl Lek Listy 2016;117(8): 472– 9.
26. Bondan EF, Martins Mde F,Bernardi MM. Propentofylline reverses delayed remyelination in streptozotocin-induced diabetic rats. Arch Endocrinol Metab 2015;59(1):47– 53. doi: S2359-39972015000100047.
27. Rezayof A, Razavi S, Haeri-Rohani A, et al. GABA(A) receptors of hippocampal CA1 regions are involved in the acquisition and expression of morphine-induced place preference. Eur Neuropsychopharmacol 2007;17(1):24– 31. doi: 10.1016/ j.euroneuro.2006.02.003.
28. Lashgari R, Motamedi F, Zahedi Asl S, et al. Behav-ioral and electrophysiological studies of chronic oral administration of L-type calcium channel blocker verapamil on learning and memory in rats. Behav Brain Res 2006;171(2):324– 8. doi: 10.1016/ j.bbr.2006.04.013.
29. Casamenti F, Di Patre PL, Bartolini L, et al. Unilateral and bilateral nucleus basalis lesions: differences in neurochemical and behavioural recovery. Neuroscience 1988;24(1):209– 15.
30. Yamada K, Komori Y, Tanaka T, et al. Brain dysfunction associated with an induction of nitric oxide synthase following an intracerebral injection of lipopolysaccharide in rats. Neuroscience 1999;88(1):281– 94.
31. Guerci B, Bohme P, Kearney-Schwartz A, et al. Endothelial dysfunction and type 2 diabetes. Part 2: altered endothelial function and the effects of treatments in type 2 diabetes mellitus. Diabetes Metab 2001;27(4 Pt 1):436– 47.
32. Nordberg J, Arner ES. Reactive oxygen species, antioxidants, and the mammalian thioredoxin system. Free Radic Biol Med 2001;31(11):1287– 312.
33. Scaccini C, Chiesa G, Jialal I. A critical assessment of the effects of aminoguanidine and ascorbate on the oxidative modification of LDL: evidence for interference with some assays of lipoprotein oxidation by aminoguanidine. J Lipid Res 1994;35(6):1085– 92.
34. Burcham PC, Kaminskas LM, Fontaine FR, et al. Aldehyde-sequestering drugs: tools for studying protein damage by lipid peroxidation products. Toxicology 2002:181– 2.
35. Jedidi I, Therond P, Zarev S, et al. Paradoxical pro-tective effect of aminoguanidine toward low-densitylipoprotein oxidation: inhibition of apolipoprotein Bfragmentation without preventing its carbonylation. Mechanism of action of aminoguanidine. Biochemistry 2003;42(38):11356– 65. doi: 10.1021/bi034539w.
36. Nilsson BO. Biological effects of aminoguanidine: an update. Inflamm Res 1999;48(10):509– 15.
37. Ates O, Cayli SR, Yucel N, et al. Central nervous system protection by resveratrol in streptozotocin-induced diabetic rats. J Clin Neurosci 2007;14(3):256– 60.
38. Celik S, Erdogan S. Caffeic acid phenethyl ester (CAPE) protects brain against oxidative stress and inflammation induced by diabetes in rats. Mol Cell Biochem 2008;312(1– 2):39– 46. doi: 10.1007/ s11010-008-9719-3.
39. Hao W, Wu XQ, Xu RT. The molecular mechanism of aminoguanidine-mediated reduction on the brain edema after surgical brain injury in rats. Brain Res 2009;1282:156– 61. doi: 10.1016/ j.brainres.2009.05.041.
40. Yildiz G, Demiryurek AT, Sahin-Erdemli I, et al. Comparison of antioxidant activities of aminoguanidine, methylguanidine and guanidine by luminol-enhanced chemiluminescence. Br J Pharmacol 1998;124(5):905– 10. doi: 10.1038/ sj.bjp.0701924.
41. Ivanova S, Botchkina GI, Al-Abed Y, et al. Cerebral ischemia enhances polyamine oxidation: identification of enzymatically formed 3-aminopropanal as an endogenous mediator of neuronal and glial cell death. J Exp Med 1998;188(2):327– 40.
42. Phillips SA, Thornalley PJ. Formation of methylglyoxal and D-lactate in human red blood cells in vitro. Biochem Soc Trans 1993;21(2):163S.
43. Di Loreto S, Caracciolo V, Colafarina S, et al. Methylglyoxal induces oxidative stress-dependent cell injury and up-regulation of interleukin-1beta and nerve growth factor in cultured hippocampal neuronal cells. Brain Res 2004;1006(2):157– 67. doi: 10.1016/ j.brainres.2004.01.066.
44. Di Loreto S, Zimmitti V, Sebastiani P, et al. Methylglyoxal causes strong weakening of detoxifying capacity and apoptotic cell death in rat hippocampal neurons. Int J Biochem Cell Biol 2008;40(2):245– 57.
45. Huang X, Wang F, Chen W, et al. Possible link between the cognitive dysfunction associated with diabetes mellitus and the neurotoxicity of methylglyoxal. Brain Res 2012;1469:82– 91.
46. Yu PH, Zuo DM. Aminoguanidine inhibits semi-carbazide-sensitive amine oxidase activity: implicat-ions for advanced glycation and diabetic complications.Diabetologia 1997;40(11):1243– 50. doi: 10.1007/ s001250050816.
47. Thornalley PJ. The glyoxalase system in health and disease. Mol Aspects Med 1993;14(4):287– 371. 48. Li Z, Zhang W, Grunberger G, et al. Hippocampal neuronal apoptosis in type 1 diabetes. Brain Res 2002;946(2):221– 31.
49. Li Z, Sima A. C-peptide and central nervous system complications in diabetes. Exp Diabesity Res 2004;5(1):79– 90.
50. Duchen MR. Mitochondria in health and disease: perspectives on a new mitochondrial biology. Mol Aspects Med 2004;25(4):365– 451. doi: 10.1016/ j.mam.2004.03.001.
51. Li ZG, Zhang W, Grunberger G, et al. Hippocampal neuronal apoptosis in type 1 diabetes. Brain Res 2002;946(2):221– 31.
52. Choi BM, Pae HO, Jang SI, et al. Nitric oxide as a pro-apoptotic as well as anti-apoptotic modulator. J Biochem Mol Biol 2002;35(1):116– 26.
53. Moncada S, Bolanos JP. Nitric oxide, cell bioenergetics and neurodegeneration. J Neurochem 2006;97(6):1676– 89.
54. Green DR, Reed JC. Mitochondria and apoptosis. Science 1998;281(5381):1309– 12.
55. Friedlander RM. Apoptosis and caspases in neuro-degenerative diseases. N Engl J Med 2003;348(14):1365– 75. doi: 10.1056/ NEJMra022366
56. Srinivasan S, Stevens M, Wiley J. evidence for apoptosis and associated mitochondrial dysfunction. Diabetic peripheral neuropathy 2000; 49:1932– 38.
Štítky
Paediatric neurology Neurosurgery NeurologyČlánok vyšiel v časopise
Czech and Slovak Neurology and Neurosurgery
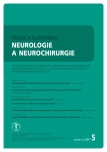
2017 Číslo 5
- Memantine Eases Daily Life for Patients and Caregivers
- Metamizole vs. Tramadol in Postoperative Analgesia
- Memantine in Dementia Therapy – Current Findings and Possible Future Applications
- Obstacle Called Vasospasm: Which Solution Is Most Effective in Microsurgery and How to Pharmacologically Assist It?
- Spasmolytic Effect of Metamizole
Najčítanejšie v tomto čísle
- Leber Hereditary Optic Neuropathy
- Essential Tremor – Is There a New Nosological Concept?
- Statin-induced Necrotizing Autoimmune Myopathy
- Invasive Methods in the Treatment of Advanced Parkinson’s Disease