Halloysite – interesting nanotubular carrier for drugs
Halloysit – zajímavý nanotubulární nosič pro léčiva
Halloysit je v přírodě se vyskytující minerál příbuzný kaolinu, který se vyznačuje specifickým tvarem částic ve formě ultramikroskopických mnohovrstevných dutých prázdných válečků. Našel své uplatnění v mnoha průmyslových odvětvích a vzhledem ke svým výhodným vlastnostem, např. biokompatibilitě, adsorpci léčiv, vysoké mechanické odolnosti a snadné dostupnosti, také ve farmacii a medicíně. Může vázat léčiva na povrchu nebo uvnitř tubulů a zvyšovat jejich stabilitu nebo měnit jejich uvolňování. Povrch tubulů je snadno modifikovatelný pro využití v transportních lékových systémech. Halloysit je perspektivním materiálem pro kostní implantáty a pro řízené uvolňování biomakromolekul. Přehledový článek se zabývá farmaceutickým a biomedicínským využitím tohoto zajímavého materiálu a zahrnuje původní experimentální práce z posledních let.
Klíčová slova:
halloysit • adsorpce léčiv • uvolňování léčiv • transportní lékové systémy • tkáňové inženýrství
Authors:
Miloslava Rabišková
Authors‘ workplace:
Department of Pharmaceutical Technology, Faculty of Pharmacy, Charles University
Published in:
Čes. slov. Farm., 2012; 61, 255-260
Category:
Review Articles
Overview
Halloysite is a naturally occurring mineral similar to kaolin, possessing a special particle shape in the form of ultramicroscopic multi-layered hollow cylinders. It is utilizable in many industrial branches and due to its advantages, e.g. biocompatibility, drug entrapment, high mechanical strength and easy natural availability also on the pharmaceutical field and in medicine. It can bind drugs on the surface or inside of tubules and increase drug stability or change its release. Surface of the tubules can be readily modified for the application in drug delivery systems. Halloysite was reported as promising material for bone implants and controlled delivery of biomacromolecules. This review is dealing with pharmaceutical and biomedical usage of this interesting material and is including original experimental work published recently.
Keywords:
halloysite • drug entrapment • drug release • drug delivery systems • tissue engineering
Introduction
Clay, as a rock term, describes a soft, loose, earthy material. Clay minerals are essentially hydrous aluminium silicates with a sheet-like structure (phyllosilicates) where magnesium or iron may substitute wholly or partly for aluminium. The most common clay minerals are kaolinite, halloysite, smectite, allophane, chlorite and illite1).
Halloysite (Al4Si4O10(OH)84H2O), named after Baron Omalius d’Halloysite, the Belgian geologist, is a well-known weathering product of pyroclastic materials. Halloysite is aluminosilicate clay mined from natural deposits in countries such as America, Brazil, China, France, Japan, South Korea and Turkey; it is chemically similar to kaolin, but differs in having a hollow microtubular rather than a stacked plate-like structure. Because of the mechanism by which halloysite is formed from amorphous allophane by long-term weathering, a small amount of this precursor may be associated with certain halloysite samples. Halloysite tubules from different regions and within samples vary in dimensions indicating the need to source halloysite from one deposit where excess variability is likely to be a critical issue. The morphology of this 1 : 1 layered mineral has been shown to be closely related to its genesis and composition2). While chemically similar to kaolin, halloysite has predominantly the shape of ultramicroscopic multi-layered hollow cylinders. Many researchers have done lot of work on the characterization of halloysite. Recently, it has been reported that halloysite has typical dimensions of 10–50 nm in outer diameter, 5–20 nm in inner diameter and the length of 2–40 μm3, 4). Halloysite’s layer silicate and the crystal structure are formed by two building blocks, i.e. sheets of corner sharing (SiO4) tetrahedral and sheets of edge sharing (AlO6) octahedral (Fig. 1). In the (SiO4) sheets the tetrahedra share three corners and the unbounded tetrahedral apices are all pointing along the same direction5). The layers building up the final structure are composed by one of each of these sheets so that the crystal structure of halloysite is described as 1 : 1 dioctahedral layer silicate. The water molecules are sitting between two consecutive layers and create slight differences in the relative orientation between neighbouring layers (Fig. 2). This might give rise to the differences in the symmetry of the final structure. This structure of halloysite elucidates the close similarity with the structure of kaolinite and experimentally it is difficult to distinguish between them. As a matter of fact, the structure of halloysite-(7 Å) is completely analogous to a disordered kaolinite structure. Tubular or cylindrical particles of different dimensions are normally found with halloysite even though some other morphology (fibers, plates etc.) has also been reported. Thus the powder X-ray diffraction patterns are rather complicated with extensive overlapping of reflections accompanied by a high background. As a result X-ray diffraction powder patterns are virtually identical for both structures. As stated, the most noticeable difference is probably the particle shape. Thus, the convention of the presence of cylindrical or tubular particles can be adopted as indicative of halloysite while samples with platy particles might be named as kaolinite6, 7). Halloysite can intercalate a monolayer of water molecules but this interlayer water is weakly held and giving a basal spacing near 10 Å to resultant nanotubes. Halloysite with (10 Å) form can readily dehydrate to give the halloysite (7 Å) form, the form very much similar to kaolinite as mentioned above. Halloysite either hydrated form or dehydrated form has a greater propensity for intercalating organic molecules8).
Halloysite is traditionally used in the manufacture of high quality ceramic white-ware. Its microcylinders are an interesting alternative geometry for the entrapment and release of various active agents in microencapsulation applications4). Therefore recently halloysite has been studied and used in many other applications especially for its shape of nanotubes. There are many patents for the use of halloysite in industry for ceramics, cements and fertilizers. It has been investigated also as the support material for catalytic convertors in exhaust systems or as a material for the extended delivery of a range of biocides following incorporation into marine antifouling paints9). Besides its enormous potential applications because of its tubulary shaped particles, halloysite can find its use as a template in nanotechnology, as a nanofiller and in controlled release technology for a range of active agents7). Examples of tubular carriers are depicted in Fig. 3.
Characterization of halloysite
As mentioned before, halloysite coming from different regions can differ not only by the diameter (and structure) but also by the composition. Therefore, the preliminary characterization of the material is necessary. Prior to its experimental use, halloysite should be characterized for particle size, intercalation, porosity and surface area. Allophane removal procedure10), zeta potential measurements, and thermogravimetric analysis should be also included into preliminary tests9). Particle size analysis can be provided by either laser diffraction or some of microscopic evaluation (scanning electron microscopy – SEM, transmission electron microscopy – TEM) using image analysis. When using SEM, samples of halloysite are mounted on aluminium stubs and vacuum coated with gold film. This technique is able to detect possible particle agglomerates, which could be eliminated using a sieve.
Zeta potential measurements on halloysite over a wide pH range indicate halloysite nanotubes charge. They exhibit slightly negative surface charge at very low pH. With increasing pH value from 2 to 6, the negative charge significantly increases to ~ 27 mV reaching a plateau at pH 6–9, before dropping further at pH values greater than 10. These values were found for halloysite obtained from New Zealand9), for halloysite mined in Portugal and some other11). The reason for the generation of the negative surface charge with increasing pH is that in the curved structure, the silica is mainly positioned on the outer surfaces of the tubule, whereas the alumina is present mainly on the inner surface and edges of the tubules. Exposure of both oxides to water causes the formation of surface hydroxyl groups that can ionize. Silica, being an acidic oxide, gives negative charge to the surface of tubules over a wide range of pH, while alumina shows a more amphoteric behavior. As a result, halloysite tends to have a polyanionic surface, except at very low pH, and should readily bind cationic drugs or coating polymers from solution9).
X-ray diffraction (XRD) techniques are used to identify halloysite and to determine whether it is present in its dehydrated (7 Å form), hydrated (10 Å form) or partially hydrated form. Dehydrated halloysite cannot be rehydrated by simple exposure of water12). For rehydration process of halloysite, the procedure of Wada (1961)13) can be used. In this method, halloysite is grounded with potassium acetate to form an intermediary intercalation complex. This complex is then rinsed with water to remove the salt resulting in hydrated halloysite. Afterwards intercalation process of small molecules containing two functional groups, preferably –OH and/or –NH2 (e.g. glycerol) through hydrogen bonding can be provided14).
Porosity and surface area measurements can be provided using mercury porosimetry9) or better by textural analysis by nitrogen adsorption-desorption technique15).
Flexibility of halloysite nanotubes was studied16) by the measurement of Young’s modulus of individual particles using TEM with a bending stage. TEM micrographs showed that halloysite nanotubules were surprisingly flexible and could be bend to almost 90º without fracture. No reduction in cross-sectional area of the bent nanotubules was observed. Therefore, it was suggested that halloysite nanotubules have a good potential to be used in high-performed structural materials, especially polymer-based nanocomposites.
Stability of halloysite nanotubules
Halloysite nanotubes are thermally stable as published in recent study16). Kadi et al. treated natural halloysite of Algerian origin at different temperatures in the range 200–1000 °C. They found that the crystal structure of halloysite is stable up to 400 °C. Thermal analysis showed that the decomposition takes place in three main steps: release of physically bounded water in the range 50–240 °C, structural decomposition through a dehydroxylation of structural aluminol groups between 480 and 640 °C and a recrystallization process at about 1000 °C. Halloysite nanotubular morphology was maintained up to 600 °C; a poorly organized structure and a progressive amorphisation of the structure were obtained between 600 and 800 °C, and at 1000 °C the nanotubes were damaged.
The long term stability of natural halloysite nanotubes was investigated at room temperature in another study17). The structural changes in purified water, acidic (1 mol/L H2SO4) and basic (1 mol/L NaOH) aqueous suspensions of halloysite samples were determined using SEM, TEM, nitrogen adsorption, XRD Raman and Fourier transformed infrared (FTIR) spectroscopy accompanied by monitoring the concentration of dissolved Si and Al in solution. It has been revealed that under acidic conditions the dissolution of halloysite is initiated on the inner surface of nanotubes leading to the formation of amorphous spheroidal nanoparticles of SiO2 whereas under basic conditions the dissolution of inner surface of nanotubules is accompanied by the formation of Al (OH)3 nanosheets.
Halloysite nanotubes as a potential drug delivery carrier
Efficient delivery of a drug requires delivering the drug at intended site with predetermined rate. Halloysite’s cylindrical structure has a potential for drug loading, particularly of cationic agents by adsorption onto its polyanionic faces or more extensively by entrapment into its hollow lumen18). Many alternatives like lipid microtubules or carbon nanotubules have similar morphology as well as similar drug loading capacity but halloysite because of its abundance and various surface properties is a good candidate for drug loading:
- Bi-layered alignment gives halloysite’s nanotubules cylindrical shape;
- The inner and outer faces of tubular walls of halloysite nanotubules carry a net negative charge functioning as polyvalent anion;
- Halloysite nanotubules with amphoteric edges give negative charge at high pH and positive charge at low pH;
- Unusual shape and charge distribution of halloysite favour face-to-edge attachment in aqueous suspensions at slightly alkaline pH and facilitate binding particularly cations to unreacted faces.
All these properties of halloysite nanotubules make halloysite a possible candidate for drug loading either with entrapping agents within the lumen of tubules using retardant polymers or by cationic coating and other approaches for prolonged release rate, or by swapping intercalated water if present for low molecular weight agents7).
Drug entrapment and release
The in vitro release characteristics of a number of drugs from halloysite have been previously examined and shown to have sustained release properties4, 18–20). Halloysite nanotubules provide dispersions at submicrone level in aqueous media. Entrapping of drug molecules in such structures is a helpful strategy for protecting the drug against the chemical as well as enzymatic degradation, for enhancement of aqueous solubility, or to reduce drug dissolution rate and target drug release.
Sustained release of diltiazem hydrochloride and propranolol hydrochloride as model drugs from halloysite nanotubules was the objective of the paper published by Levis and Deasy18). In this investigation, highly soluble cationic drug, diltiazem HCl was shown to bind to the polyanionic surfaces of the tubules and thus a slight prolonged release effect on drug dissolution was achieved due to reversible chemisorption and/or hindered release from the drug loaded lumen. More prolonged release effect was apparent when the less water soluble cationic drug, propranolol HCl, was examined. Attempts to further delay drug release by loading diltiazem HCl from a povidone solution into the halloysite had little effect. However, a range of cationic polymers, including chitosan cross-linked with glutaraldehyde or Eudragit E®, was shown to bind to halloysite and was used to achieve significantly slow drug release. Coating with adequate polyethyleneimine was particularly effective at prolonged drug release, being dependent on the architecture of the interaction between the polycation and the mineral. When a range of alkyl-2-cyanoacrylate monomers applied from a non-aqueous solvent by an in situ polymerization procedure was examined, diltiazem HCl loaded halloysite dispersed in poly-iso-butyl cyanoacrylate was found the most effective at reducing the burst effect noted with aqueous coating systems.
The entrapment of hydrophilic and lipophilic drugs into halloysite nanotubules was reported in another study4). Oxytetracycline hydrochloride, as a representative of highly soluble hydrophilic drug, has been entrapped alone and in the blend with compatible polymer – epoxy Quitol 651 to reduce the burst effect of drug release within the interval of 30 h from 20% to ~ 5%, respectively. The entrapment of khellin, a hydrophobic vasodilator, required a different approach. In this case, the halloysite was treated with ethylene glycol, so that the clay would wet and permit entrapment of the hydrophobic khellin. The neat khellin was entrapped in a melted lipid state and then allowed to solidify in the clay. Its release was very slow, as expected, due to drug’s low solubility in water. For entrapment of nicotinamide adenine dinucleotide (NAD), it was found necessary to add a water based polymer, povidone, in order to increase the viscosity of the solution to aid in NAD retention. NAD release was prolonged to approx. 12 h. This way, successful entrapment of both hydrophilic and hydrophobic drugs after appropriate pre-treatment of the clay can be achieved.
Viseras et al.21) studied 5-aminosalicylic acid (5-ASA) adsorption onto halloysite. The 5-ASA adsorption was explained as the result of two separate processes, i.e. initial rapid adsorption of 5-ASA on the external halloysite surface and following slow adsorption of the drug inside the phyllosilicate pores. Authors stated 5-ASA/ halloysite as promising delivery system.
Topic utilization of halloysite for cosmetic applications was reported recently by Suh et al.22) Natural halloysite mined in USA and New Zealand, respectively, was investigated as a nanocontainer for the loading and prolonged release of glycerol in the function of moisturizing agent. It was found that glycerol release from nanotubes exceeded 20 h and halloysite from USA had significantly higher loading capability than halloysite from New Zealand.
A novel drug delivery system for the treatment of periodontitis was developed using two components. The first was tetracycline base loaded into the microtubular excipient halloysite, which was coated with chitosan to further retard drug release. Encapsulation efficiencies of 32.5% were achieved. The second component developed was a vehicle for the drug loaded coated halloysite, which was primarily based on the thermoresponsive polymer, poloxamer 407 supplemented by polyethelene glycol 20,000 and octyl cyanoacrylate so that the mobile product at room temperature would gel with the temperature rise following syringing into a periodontal pocket. The final formulation was stable for at least 9 months (room temperature) showing prolonged release of the antibiotic up to 6 weeks20).
Pellets composed of halloysite and microcrystalline cellulose were prepared with the aim of producing a drug delivery vehicle for prolonged release of the opioid fentanyl with low risk for dose dumping at oral intake of the highly potent drug. Drug release profiles of intact and crushed pellets, to simulate swallowing without or with chewing, in pH 6.8, pH 1, and in 48% ethanol were recorded in order to replicate the conditions in the small intestines, in the stomach, as well as cointake of the drug with alcohol. Prolonged release for ~ 3–4 h was obtained in all studied solutions from intact pellets, whereas crushed pellets released the drug content during ~ 2–3 h. The finding that prolonged release profile could be obtained both in alcohol and after crushing of the pellets, shows that the ceramic carrier under investigation, at least to some extent, hampers dose dumping, and may thus be a promising material in future developments of new opioid containing oral dosage forms23).
Halloysite utilization in nanotube-targeted delivery of doxorubicin to circulating tumor cells was announced in the paper of Mitchell et al.24) The presence of circulating tumor cells (CTCs) is believed to lead to the formation of secondary tumors via an adhesion cascade involving interaction between adhesion receptors of endothelial cells and ligands on CTCs. Many CTCs express sialylated carbohydrate ligands on their surfaces that adhere to selectin protein found on inflamed endothelial cells. In this work, the feasibility of using immobilized selectin proteins as a targeting mechanism for CTCs under flow was investigated. Herein, targeted liposomal doxorubicin (L-DXR) was functionalized with recombinant human E-selectin (ES) and polyethylene glycol (PEG) to target and kill cancer cells under shear flow. Healthy circulating cells such as red blood cells were not targeted by this mechanism and were left to freely circulate, and minimal leukocyte death was observed. Halloysite nanotube HNT-coated microtube devices immobilized with nanoscale liposomes significantly enhanced the targeting, capture, and killing of cancer cells. The advantage of this device is that by immobilizing liposomes onto the microtube surface, the potential distribution of targeted L-DXR into circulation is reduced and a lower dose of the drug is necessary. The proposed device demonstrates potential application of HNT-coated microtubes for reducing the probability of metastasis, and suggests new strategies for enhancing targeted delivery of chemotherapeutics to CTCs.
Grafted halloysite
Halloysite was described in many articles as very promising material for applications in the fields of nanocomposites, enzyme immobilization, and controlled release because of its advantages including unique tubular structure, rich functionality, good biocompatibility, high mechanical strength and easy natural availability. Biocompatibility of halloysite nanotubules can be further improved by non-covalent functionalization with biomolecules, e.g with DNA or amylose25, 26).
Functionalization of halloysite nanotubes by grafting with γ-aminopropyltriethoxysilane (APTES)27) is useful for improving the properties of halloysite for these applications. The surface chemistry of halloysite nanotubes is reported as readily modified; the mechanism of modification includes not only the direct grafting of APTES onto the surface hydroxyl groups, but also oligomerization forming a cross-linked structure. Some APTES may also bridge via hydrogen bonds. The results show that the modification methods and the raw tubular halloysite sample must be tailored to meet the requirements of a specific application. Another example of grafted halloysite nanotubes was published by Mu et al.28) The paper is describing preparation and characterization of halloysite nanotubes grafted hyperbranched (co)polymers via surface-initiated self- condensing vinyl (co)polymerization. The method was found to be utilizable for grafting the surface of halloysite with different components.
Halloysite nanotube based gene delivery system was explored for loading and intracellular delivery of antisense oligonucleotides, in which functionalized halloysite nanotubes with γγ-aminopropyltriethoxysilane were used as carriers and antisense oligonucleotides as therapeutic gene for targeting survivin (a protein inhibiting apoptosis), which is selectively overexpressed in most human cancers, but not in normal tissues. In vitro cytotoxicity results reported that functionalized halloysite nanotubes complexes could efficiently improve intracellular delivery and enhance antitumor activity of antisense oligonucleotides29).
Halloysite nanotubes for tissue engineering
Recently, nanotubes are being used also for tissue engineering. Tissue engineering expects the compatibility of nanotubes with biological matrix and therefore halloysite seems as promising material as it is biocompatible with cellular organelles. Its low cost is also an important benefit. As alumina and silica groups are located on the surfaces of halloysite nanotubules especially on their crystal edges: the formation of hydrogen bonding interactions between halloysite and biological components is easy making halloysite an ideal candidate for bionanomaterial and bionanocomposite films.
Biocompatibility of halloysite is essential for its potential application in biopolymer composites, bone implants, controlled delivery of biomolecules, and for in vivo protecting coatings. Several published studies30) indicate that halloysite exhibits a high level of biocompatibility and very low cytotoxicity. It can improve the properties of several biodegradable and biocompatible polymers as well. Poly vinyl alcohol (PVA), for instance, possesses many desirable properties important for biomedical applications, but its high hydrophilicity limits its use in living systems. To improve its biological applications as well as mechanical properties PVA based bionanocomposites were designed. Halloysite/PVA nanocomposite films have shown its potential application in bone tissue engineering and drug delivery systems. Halloysite/PVA bionanocomposite films were characterized by cell culture studies. Cell morphological studies showed that modified film increases the cell adhesion and cell in vitro growth31).
Conclusion
As mentioned, halloysite nanotubes have immense biological and non-biological applications; some of them are becoming very important including halloysite as a component for fabrication, corrosion prevention, polymerization, immobilization, thermostability, drug delivery or tissue engineering. The objective of this review was to introduce halloysite potential on the field of pharmacy and medicine. In near future, it is expected its practical use in controlled drug release as outlined in the case of few model drugs reported here. The drugs which can be entrapped in halloysite nanotubes are described usually to have a cationic nature. In the following original article, we would like to present the possibility to entrap anionic model drug into halloysite nanotubules.
Conflicts of interest: none.
Received 5 November 2012 / Accepted 14 November 2012
Address:
prof. PharmDr. Miloslava Rabišková, CSc.
Department of Pharmaceutical Technology,
Faculty of Pharmacy, Charles University
Heyrovského 1203, 500 05 Hradec Králové
e-mail: rabiskova@seznam.cz
Sources
1. Christie T., Thompson B., Brathwaite B. Mineral commodity report 20. Clays New Zealand Mining. 2000; 27, 26–43.
2. Adamo P., Violante P. Wilson M. J. Tubular and spheroidal halloysite in pyroclastic deposits in the area of the Roccamonfina volcano (Southern Italy). Geodema 2001; 99, 295–316.
3. Christie T., Fletcher W. K. Contamination from forestry activities: implications for stream sediment exploration programmes. Journal of Geochemical Exploration 1999; 67, 201–210.
4. Price R. R., Gaber B. P., Lvov Y. M. In-vitro release characteristics of tetracycline HCl, khellin and nicotinamide adenine dinucleotide from halloysite. J Microencapsulation 2001; 18(6), 713–722.
5. Joussein B., Petit D., Churchman B., Theng J., Righi S., Delvaux E. Halloysite clay minerals. Clay Miner. 2005; 40, 383–426.
6. Klimkiewicz R., Drag, E. B. Catalytic activity of carbonaceous deposits in zeolite from halloysite in alcohol conversions. J. Phys. Chem. Solids 2004; 65, 459–464.
7. Rawtani D., Agrawal Y. K. Multifactorious appliactions of halloysite nanotubes. Rev. Adv. Mater. Sci. 2012; 30, 282–293.
8. Joussein E., Petit S., Delvaux B. Behavior of halloysite clay under formamide treatment. Applied Clay Science 2007; 35, 17–24.
9. Levis S. R., Deasy P. B. Characterization of halloysite for use as a microtubular drug delivery system. Int. J. Pharm. 2002; 243, 125–134.
10. Kirkman J. H. Halloysite discs and cylinders. Clay Miner. 1977; 12, 199–216.
11. Tari G., Bobos I., Gomes C. S. F., Ferreira J. M. F. Modification or surface charge properties during kaolinite to halloysite 7 Å transformation. J. Colloid Interf. Sci. 1999; 210, 360–366.
12. Harrison J. L., Greenburg S. S. Dehydration of fully hydrated halloysite from Lawrence county Indiana. Clay Miner. 1962; 9, 374–377.
13. Wada K. Lattice expansion of kaolinite minerals by treatment with potassium acetate. Am. Miner. 1961; 46, 78–91.
14. Carr R. M. Chih H. Complexes of halloysite with organic compounds. Clay Miner. 1971; 9, 153–166.
15. Kadi S., Lellou S., Marouf-Khelifa K., Schott J., Gener-Batonneau I. Preparation, characterization and application of thermally treated Algerian halloysite. Microporous and Mesoporous Materials 2012; 158, 47–54.
16. Lu D., Chen H., Wu J., Chan C. M. Direct measurements of the Young´s modulus of single halloysite nanotube using a transmission electron microscope with a bending stage. Journal of Nanoscience and Nanotechnology 2011; 9, 7789–7793.
17. White R. D., Bavykin D. V., Waish F. C. The stability of halloysite nanotubes in acidic and alkaline aqueous suspensions. Nanotechnology 2012; 23 (6), DOI: 10. 1088/0957-4484/23/6/065705
18. Levis S. R., Deasy P. B. Use of coated microtubular halloysite for the sustained release of diltiazem hydrochloride and propranolol hydrochloride. Int. J. Pharm. 2003; 253, 145–157.
19. Lvov Y. M., Price R., Gaber B., Ichinose I. Thin film nanofabrication via layer-by-layer adsorption of tubule halloysite, spherical silica, proteins and polycations. Colloids and Surfaces A: Physicochem. Eng. Aspects 2002; 198-200, 375–382.
20. Kelly H. M., Deasy P. B., Ziaka E., Claffey N. Formulation and preliminary in vivo dog studies of a novel drug delivery system for the treatment of periodontitis. Int. J. Pharm. 2004; 274, 167–183.
21. Viseras M. T., Aguzzi C,. Cerezo P., Viseras C., Valenzuela C. Equilibrium and kinetics of 5-aminosalicylic acid adsorption by halloysite. Microporous and Mesoporous Materials 2008; 108, 112–116.
22. Suh Y. J., Kil D. S., Chung K. S., Abdullayev E., Lvov Y. M., Montagyt D. Natural nanocontainer for the controlled delivery of glycerol as moisturizing agent. Journal of Nanoscience and Nanotechnology 2001; 11(1) 661–665.
23. Forsgren J., Jamstorp E., Bredenberg S., Engqvist H., Strømme M. A ceramic drug delivery vehicle for oral administration of highly potent opioids. J. Pharm. Sc. 2010; 99(1), 219–226.
24. Mitchell M. J., Chen C. S., Ponmudi V., Hughes A. D., King M. R. E-selectin liposomal and nanotube-targeted delivery of doxorubicin to circulating tumor cells. J. Controlled Release 2012; 160, 609–617.
25. Zhou W.Y., Guo B., Liu M., Liao R., Rabie B.M., Jia D. Poly(vinylalcohol) halloysite nanotubes bionanocomposite films: Properties and in vitro osteoblasts and fibroblasts response. Journal of Biomedical Materials Research Part A 2010; 93, 1574–1587.
26. Zhang L., Wang T., Liu P. Polyaniline-coated halloysite nanotubes via in situ chemicla polymerazation. Applied Surface Science 2008; 2555, 2091–2097.
27. Yuan P., Southon P. D., Liu Z., Green M. E. R., Hook J. M., Antill S. J. Kepert C. J. Functionalization of halloysite clay nanotubes by grafting with γ-aminopropyltriethoxysilane. J. Phys. Chem. 2008; 112, 15742–15751.
28. Mu B., Zhao M., Liu P. Halloysite nanotubes grafted hyperbranched (co)polymers via surface-initiated self- condensing vinyl (co)polymerization. J .Nanopart. Res. 2008; 10, 831–838.
29. Shi Y. F., Tian Z., Zhang Y., Shen H. B., Jia N. Q. Functionalized halloysite nanotube-based carrier for intracellular delivery of antisense oligonucleotides. Nanoscale Research Letters 2011; 6, 608–615.
30. Vergaro V., Abdullayev E., Lvov Y. M., Zeitoun A., Cingolani R., Rinaldi R., Leporatti S. Cytocompability and uptake of halloysite clay nanotubes. Biomacromolecules 2010; 11, 820–826.
31. Cavallaro G., Lazzara G., Milioto S. Dispersions of nanoclays of different shapes into aqueous and solid biopolymeric matrices. Extended physicochemical study. Langmuir 2011; 27(3), 1158–1167.
Labels
Pharmacy Clinical pharmacologyArticle was published in
Czech and Slovak Pharmacy
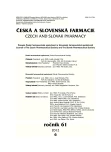
2012 Issue 6
Most read in this issue
- Evaluation of content uniformity of tablets with a low content of the active ingredient with a narrow therapeutic index
- Analysis of pharmaceutical care in dispensing of over-the-counter orlistat
- Halloysite – interesting nanotubular carrier for drugs
- INSTRUCTIONS FOR THE AUTHORS SUBMITTING PAPERS TO THE JOURNAL CZECH AND SLOVAK PHARMACY