Current concepts in cerebral oximetry for adult cardiac surgery
Současný koncept mozkové oximetrie v kardiochirurgii dospělých
Přes významné pokroky v chirurgické léčbě a operační technice se závažné pooperační komplikace vyskytují u 2–5 % kardiochirurgických pacientů. Cerebrální oxymetrie nabízí možnost okamžitého monitorování v situacích, které mohou vést k poškození mozkové tkáně. Přes nové pokroky vinfra-neared spektrometrii a získané informace z klinických studií, neprobíhá zavádění do klinické praxe tak rychle, jak se očekávalo. Důvodem jsou a) rozdíly mezi výzkumem modelových sitacích a použitím v biologických tkáních; b) chyby při obsluze; c) pohybové artefakty a multidistanční měření a d) rozdíly mezi jednotlivými přístroji. Přehledový článek popisuje použití mozkové oxymetrie u dospělých kardiochirurgických pacientů, ukazuje na přednosti a nedostatky metody, shrnuje hlavní vlastnosti nejpoužívanějších přístrojů a poskytuje aktuální přehled literárních údajů.
Klíčová slova:
neurologický výsledek – pooperační komplikace – mozková oxymetrie – kardiochirurgie
Authors:
Javier G. Castillo 1; Amanda J. Rhee 2; George Silvay 2
Authors place of work:
Department of Cardiothoracic Surgery, The Mount Sinai Medical Center, New York, NY
1; Department of Anesthesiology, The Mount Sinai Medical Center, New York, NY
2
Published in the journal:
Anest. intenziv. Med., 23, 2012, č. 5, s. 242-252
Category:
Anesteziologie - Přehledový článek
Summary
Despite significant impovements in surgical management and perioperative techniques, adverse neurological outcomes still remain a serious postoperative complication in patients undergoing cardiac surgery with an estimated incidence that ranges from 2% to 5% in most centers. Cerebral oximetry has been postulated as an emerging tool for real-time detection of clinical scenarios that can potentially predispose to cerebral injuries. Despite recent advances in near-infrared spectroscopy and an increasing availability of clinical data, the application of cerebral oximetry has not been as rapid as expected due to several reasons such as: a) disparities between research models and biological tissues, b) management errors, c) variations in susceptibility to movement artifacts or multidistance measurements and d) discrepancies among devices. This systematic review critically evaluates the application of cerebral oximetry in adult cardiac surgical patients, addresses its major technological strengths and limitations, summarizes the main characteristics of widely used devices, and provides an overview of the most recent literature.
Key words:
neurologic outcome – postoperative complication – cerebral oximetry – cardiac surgery
Introduction
Despite significant improvements in anesthetic management, surgical techniques, and perioperative care (Figure 1) of patients undergoing cardiac surgery, adverse cerebral outcomes still remain a serious postoperative complication with an estimated incidence that ranges from 2% to 5% in most centers [1, 2]. Thus, in order to decrease postoperative neurological morbidities, research efforts are being focused on the achievement of real-time detection of scenarios that may potentially predispose to brain injuries [3].
The first attempt to directly monitor cerebral oxygenation was performed by catheterization of the jugular bulb given a fairly possible association between jugular venous bulb oxygen saturation and postoperative cognitive decline [4]. However, this method is invasive, technically demanding, and requires a continuous blood flow pattern in order to provide reliable monitoring. As a result, the introduction of a non-invasive option able to procure the same information became highly desirable. This spurred the advent of cerebral oximetry (CO) as an emerging tool to assess cerebral oxygen saturation (ScO2) [5].
Since ScO2 may be used as an index of cerebral hypoperfusion [6], near-infrared spectroscopy (NIRS; a means of monitoring ScO2) might be consequently able to provide information on the imbalance between oxygen delivery and consumption throughout the entire surgical procedure [7]. Very recent advances in NIRS technology, including an easier management and applicability, have resulted in an increasing availability of clinical data. However, the progress and widespread application of NIRS has not been as rapid as expected due to several reasons such as: a) disparities between physical research models and biological tissues [8], b) errors from incorrect placement of sensors [9], c) the different degree of susceptibility to movement artifacts or multidistance measurements [10] and d) discrepancies among devices [11]. In addition, the evaluation of any new technology, such as CO, mandates the creation of expensive trials in order to elucidate the device’s range of clinical application and limitations [12].
This systematic review critically evaluates the application of CO in adult cardiac surgical patients, addresses its major technological strengths and limitations, summarizes the main characteristics of widely used devices, and provides an overview of the most recent literature.
Near-Infrared Spectroscopy
Near-infrared spectroscopy is designed to provide continuous non-invasive monitoring of cerebral metabolism [13]. Like pulse oximetry, NIRS applies the principles of light transmission and absorption to estimate the ratio of oxyhemoglobin to total hemoglobin (Hb), under the assumption that no significant dyshemoglobins are present [14]. Based on the Beer-Lambert law, which describes the behavior of light through a substance, light absorption occurs at specific wavelengths determined by the molecular properties of biologic tissues [15]. Below 700 nm, Hb demonstrates intense absorption of light, hindering light transmission, whereas above 1300 nm, water is able to entirely absorb all light particles involved in the transmission. In the 700 nm to 1300 nm range, near- -infrared light penetrates cerebral tissue to a depth of a few centimeters. Consequently, oxygenated and deoxygenated Hb behave as independent chromophores (color emission after light absorption), having different characteristics for absorption of near-infrared light wavelengths [16].
The NIRS brain monitoring system can be easily applied to the forehead of the patient with two adhesive patches [17]. A light-emitting diode (LED) generates a light beam that penetrates through the tissue and is then detected by one or more photodetectors [18]. Although based on a simple physical principle, the light scattering (intensity loss) secondary to the refractive variation of biological tissues makes the absolute quantification of cerebral tissue oxygenation a challenge [19]. In this regard, the further creation of spatially resolved spectrophotometry technology allows for the interrogation of an absolute, one-time tissue oxygen index value, which is not affected by the different path-length factors of biological tissues [20]. This theoretically eliminates vagaries associated with having to establish an accurate baseline with which to compare collected measurements to.
As with every developing technology, healthy skepticism should be maintained. The Food and Drug Administration did not require cerebral oximeters to show an outcome improvement in order to be approved. Thus, even though some may consider these monitoring devices to be the current standard of care for cardiac surgery, they have been introduced into the market without the level of evidence demanded by other therapies [21]. This paucity of evidenced based outcome data has led some to be reluctant to adopt this new technology while others have advocated their use [22]. We must continue to evaluate the validity of NIRS technology as evidence based outcome studies are completed.
Device-specific features
INVOS
The INVOS regional cerebral oximeter (Covidien, Boulder, CO) uses two wavelengths of near-infrared spectroscopy light (NIRL; 730 nm and 810 nm). The device calculates and displays the percent value of regional oxygen saturation (rSO2) as a ratio of oxygenated Hb to total Hb. Each disposable sensor contains a LED and two photodetectors which are located at a distance of 3 cm and 4 cm away from the light source in order to avoid light scattering. The proximal photodetector receives scalp tissue measurements, while the distal photodetector measures the saturation of deeper brain tissue. The first detector signal is subtracted from that of the distal detector in order to eliminate extracerebral signals. Internal software calculates then rSO2 values. The INVOS device provides rSO2 trend monitoring (changes in oxygen saturation from a predetermined or pre-anesthesia induction baseline value). Thus, inaccurate or unknown pre-anesthesia induction baseline values may result in situations where data is difficult to interpret making it an unreliable marker with which to base clinical decisions or interventions on (Figure 2).
EQUANOX
The EQUANOX regional oximeter (Nonin Medical Inc. Minnesota, MN) also measures rSO2 utilizing a dual emitter-detector sensor. To date, previous oximeters had routinely used a single light emitter and two photodetectors; and the value of the shorter path (extracranial oxygenation) was subtracted from the value of the longer path (intracranial and extracranial oxygenation). Consequently, any surface and shallow tissue variation between the two detectors could bias the final value. The novel application of two emitters and two detectors in each sensor may cancel out these tissue variations, possibly improving the accuracy of measurements. In this case, the device uses three wavelengths of NIRL (730, 810, and 880 nm) with a 2 cm distance between photodetectors (see Figure 2).
FORE-SIGHT
The FORE-SIGHT absolute cerebral oximeter (CAS Medical Systems, Branford, CT) is a continuous wave, spatially resolved (SRS), near-infrared spectrometer that was FDA approved for interrogation of absolute ScO2, a more specific description of rSO2.The device has two channels for bilateral brain monitoring. The disposable sensor has a fiber optic light source and two photodetectors at fixed distances of 1.5 cm and 5 cm respectively. Since previous research has determined that at least four different wavelengths might be required to precisely assess ScO2 (two additional photodetectors might compensate light scattering and avoid interferences from background light absorbers) the light (< 1 nm) is projected in four determined wavelengths at 690, 780, 805, and 850 nm. Contrary to the Beer-Lambert equation, those values derived from the FORE-SIGHT are not affected by the path-length factors of biological tissues due to the SRS technology. The SRS technology combines the multi-distance measurements of optical attenuation making it possible to calculate absolute cerebral saturations. The FORE-SIGHT’s capacity to estimate absolute values may enable the anesthesiologist to establish more accurate threshold values with which to trigger clinical interventions since no pre-induction or baseline assessment is necessary (see Figure 2).
NIRO
The NIRO near-infrared spectrophotometer (Hamamatsu Photonics K.K, Shizuoka, Japan) uses four wavelengths of NIRL (775, 825, 850, and 904 nm). The system has not been approved by the FDA. Its sensors contain a laser diode and three light photodetectors placed 4 cm to 5 cm away from the light source. Like the FORE-SIGHT, the NIRO also incorporates SRS technology to calculate an absolute, one-time tissue oxygenation value (see Figure 2).
Applicability of near-infrared spectroscopy
Since it is well known that cerebral oxygen demand is significantly higher than systemic oxygen demand [23], methods that assess systemic oxygenation (e. g. peripheral pulse oximetry) may not accurately reflect cerebral oxygenation and by definition, episodes of cerebral hypoxia [24]. Data has shown that mixed venous oxygen saturation does not accurately correlate with cerebral perfusion, making it challenging to define a safe range of systemic oxygenation values that correlate with adequate oxygenation of the cerebral cortex [25, 26].
Deleterious clinical situations such as improper positioning of the head with extreme left sided turning [27], misplacement of a catheter [28] or cannula leading to flow obstructions [29, 30], hypocapnia [31], or low perfusion pressures [32] have been reported to be successfully detected by CO [33–35]. This becomes particularly important when performing beating heart surgery [36], since the incidence of poor cerebral perfusion has been shown to be nearly twofold higher than in procedures performed under cardiopulmonary bypass [37]. By the same token, CO can be shown to demonstrate improved perfusion by means of a clinical intervention triggered by low SCO2 values [38].
A recent study by Heringlake et al. showed that low preoperative ScO2 values were associated with an adverse perioperative course [39]. The authors explored the applicability of ScO2 for risk stratification and compared its performance to the additive EuroSCORE. Data from an analysis of 1178 consecutive patients undergoing on-pump cardiac surgery (49% isolated CABG) demonstrated that non- -survivors (30-day-mortality) had significantly lower ScO2 values than survivors (median 58% vs. 64%). Additionally, an operating curve analysis revealed an area-under-the-curve of 0.71 and 0.77 for ScO2 and the additive EuroSCORE respectively. The authors concluded that ScO2 levels (< 50%) might be reflective of the severity of cardiopulmonary dysfunction, associated with short- and long-term mortality and morbidity, and might add to preoperative risk stratification in patients undergoing cardiac surgery.
A comparison among ScO2, radial artery oxygen saturation, and jugular bulb saturation is currently in progress by MacLeod and colleagues. Their previous work includes a head-to-head comparison of the FORE-SIGHT and INVOS devices as well as other surrogates for measuring cerebral perfusion [40]. In this study, they included healthy adult subjects who received oxygen/air gas mixtures at five minutes intervals (21%, 8%, 21%, and 50% inspired oxygen) while maintaining oxygen saturations of > 70%. After analysis, the authors contrasted the reliability of CO and reported the superiority of those devices able to measure ScO2.
Additionally, CO has been validated through a comparison with pulse oximetry, revealing that the latter detects changes in oxygenation significantly later [41]. In this study conducted by Tobias et al., the authors demonstrated that when the SaO2 decreased by 5%, the rSO2 had decreased by 16 ± 4%. In addition, the time for a 10% decrease of the rSO2 was 138 ± 29 seconds versus 189 ± 64 seconds for a 10% decrease of the SaO2.
Standarized baseline values
Numerous publications have reported data on the influence of patient demographics on ScO2 readings [42]. In a recent study of 111 patients, Kishi et al. did not find any significant correlation between ScO2 values and gender, weight, or height [43]. However, significant variations were observed between ScO2 values when age and hemoglobin concentration were taken into consideration (cerebral blood flow, volume and hemoglobin oxygenation decline exponentially with age). Additionally, the authors were able to find low values of ScO2 as a consequence of low Hb concentration.
It is of interest to know that similar results have been traditionally described in situations of volume depletion secondary to blood loss, hemodilution by cardiopulmonary bypass, or blood transfusion [44, 45]. Although it remains unclear, it seems that in the setting of volume depletion [46], the cerebral cortex might experience an important increase in oxygen extraction, resulting in a decrease in oxygenated Hb and ScO2 values [47]. These metabolic changes increase the number of photons being detected and consequently allow them to cover greater distances through the brain, resulting in an increased optical path length [48].
After analyzing patient demographics, the assessment of baseline values should be adapted to the device characteristics for optimal results. Cerebral oximetry monitoring should ideally be initiated during the anesthetic pre-induction period, especially when using regional oximeters (the need for baseline values when using absolute ScO2 remains debatable) [49]. Adhesive patches containing the light emitting source and sensors are applied bilaterally high on the frontal eminence, approximately 2 to 3cm superior to the orbital ridge to avoid the frontal sinuses. Such placement protocol is designed to avoid hair and melanin light absorption [50].
The difficulty in establishing accepted standardized baseline values for ScO2 and defining an accurate intervention threshold is partially due to the lack of standardization among devices [51–53]. Current normal ScO2 values for adult elective cardiac surgical patients are thought to be between 60% and 75% [54]. The individual and disease-specific baseline variation may be as high as 10% (67 ± 10 units) and the mean and median right versus left sensor differences has been observed to be greater than 10 units in less than 5% of the patients [55]. Therefore, from a statistical point of view, awake cardiac surgical patients with ScO2 values < 50% or a right to left difference of > 10% comprise < 5% of the general population and may be considered abnormal (Figure 3).
Intervention strategies based on cerebral oximetry
In prior years, conflicting data between the few number of clinical series and the amount of anecdotal case reports on the efficacy of CO led to its recognition as a technology with promising clinical potential [56]. However, the first randomized trials to truly elucidate the clinical scope of CO, if any, have been published during the last 3 years. Murkin and colleagues randomized 200 patients who underwent CABG to either intraoperative rSO2 real-time active monitoring or blinded rSO2 monitoring both using the INVOS cerebral oximeter [57]. Additionally, an intervention strategy based on optimizing the factors that potentially impact the cerebral poise between oxygen supply and demand (mechanical problems, mean arterial pressure, cardiac output, systemic oxygen saturation, partial pressure of carbon dioxide, cerebral metabolic rate, blood pH, body temperature, and hemoglobin abnormalities) [58] was applied to maintain the cerebral saturation values within 75% of the baseline reading. Baseline values were obtained while the patient was awake and resting comfortably with supplemental oxygen. During the surgical procedure, the intervention algorithm was triggered by an abnormal rSO2 value defined as a bilateral or unilateral 20% decrease or an absolute desaturation below 50%. Figure 4 highlights the most common therapeutic actions taken by Murkin et al. to avoid a decrease in rSO2.
The authors also reported on the use of propofol and cooling to further suppress cerebral oxygen consumption as supplemental rescue maneuvers. After data analysis, they demonstrated that monitoring cerebral rSO2 in patients undergoing CABG may minimize periods of cerebral desaturation, showing a trend towards an improvement in postoperative neurologic outcomes as well as a reduction in major organ dysfunction. This management algorithm and its applicability were updated by the same group of investigators. The authors reported the usefulness of the algorithm to reverse drastic drops in cerebral oxygen saturation in 239 consecutive patients undergoing complex cardiac procedures [59].
Slater et al. designed a prospective randomized trial in 265 patients undergoing CABG in an effort to elucidate the relationship between intraoperative cerebral oxygen desaturation and postoperative neurocognitive dysfunction [60]. Neurocognitive testing was performed preoperatively, at hospital discharge, and 3 months after. This study adopted the interventional protocol utilized by Murkin et al., and calculated a rSO2 desaturation score by multiplying rSO2 values of ≤ 50% by time in seconds. Although there was no difference between groups in terms of the incidence of neurocognitive dysfunction, patients with a score greater than 3000%-second (prolonged brain desaturation below 50%) had a higher risk of early postoperative cognitive decline and a near threefold increased risk of prolonged length of stay. After multivariate analysis, the reduction in cognitive decline in the intervention cohort was not statistically significant. According to the authors, this was possibly due to lack of adhesion to the protocol when an intraoperative rSO2 desaturation was observed. Interestingly, the positive correlation between the rSO2 desaturation score and increased length of stay may support the interpretation of CO as a surrogate of overall organ perfusion and function.
Our institutional experience with the FORE- -SIGHT cerebral oximeter has led to several publications, which reported that CO can be used as a guide for patient management during cardiac surgery. In this regard, Fischer et al. have published our extensive experience in patients undergoing deep hypothermic circulatory arrest [61]. The researchers first reported an unexpected rSO2 pattern in a patient undergoing an ascending aortic aneurysm repair [35]. In this case, despite steady hemodynamics (mean arterial pressure 50 mmHg), a decline in oxygen saturation was observed after cooling. This finding, attributed to the loss of cerebral autoregulation, suggests the need for an individualized anesthetic management, as well as the mandatory application of cerebral oximetry to provide the physician with additional guidance with which to solve perioperative complications. Moreover, the same group of investigators recently released a mathematical model to describe and interpret oxygen desaturation in this group of patients [62]. Cerebral desaturation curves from 36 patients undergoing aortic surgery with DHCA were used to create a non-linear mixed model that assumes that the rate of oxygen decline is greatest during the initial perioperative period before becoming constant. After analysis, the authors validated (± 3 minutes) two statistical models, the first one to describe cerebral desaturation and the second one to predict cerebral desaturation 15 minutes after DHCA (see Figure 4).
Clinical implications
Trends toward improvement in clinical outcomes are always subordinated to the development of relevant interventional strategies [63]. In this regard, the available interventional algorithms in CO are in an early stage and have not been corroborated by larger clinical trials. In fact, several issues will need to be solved in the near future in order to advance in the clinical applicability of CO including: a) clarification of the relevant differences between trend and absolute saturation monitoring and their clinical impact, b) validation and corroboration of interventional algorithms and scores, c) identification of those modifiable clinical variables with a potential impact on cerebral oxygen delivery and, d) evaluation of the patient management strategies used to optimize cerebral oxygen delivery.
Consequently, there are still a limited number of situations where the relationship between CO and clinical outcome is important, such as its use for monitoring the symmetry of blood flow during antegrade selective cerebral perfusion and its ability of CO to predict and avoid serious perioperative complications [64]. Presently, most of the literature on the avoidance of catastrophic events secondary to the application of CO is represented by pediatric case studies [65, 66].
Pros and Cons of cerebral oximetry
During the last decade, the increasing clinical experience with the use of CO has led to a literature debate between supporters and detractors of this technology. In this regard, it seems imperative that prospective randomized trials continue to be performed in order to further examine this technology as it evolves [67–69]. The most reported clinical advantages of using CO include: a) improved neurological outcomes (lower incidence of cerebrovascular accidents and postoperative neurocognitive decline) [70, 71], b) reduced major organ dysfunction [72], c) shortened length of stay, d) reduced surgical morbidity and mortality by avoiding catastrophic events.
In order to offer clinical benefits, CO should provide the anesthesiologist with accurate measurements that can guide clinical intervention to make a difference in surgical outcomes [73]. However, many technical and interpretive issues remain unsolved such as the lack of consensus in establishing a normal range of ScO2 values [74]. This disparity of numbers is also due in part to the absence of standardization among cerebral oximeters. Dullenkopf and coworkers compared the performance of two different oximeters (INVOS vs. NIRO) in a pediatric cohort of patients and found vague agreement of CO values between both devices [74]. Accordingly, others have published validation studies with normal healthy patients with similar results when interpreting rSO2 values from the INVOS, making it very difficult to establish or define normal baseline values [75]. However, the most surprising data came from an autopsy study where the investigators showed that despite the absence of blood flow, oximeters were able to register near-normal values [76]. These publications question the validity of CO as well as casting doubt on its impact on surgical outcomes [77].
Currently, a 20% decline in ScO2 from the patient’s baseline reading is considered abnormal in agreement to the most updated literature, thus triggering the application of interventional strategies [78, 79]. However, some of the interventional studies added to establish this reference value were inappropriately designed. In this matter, Yao et al. concluded that cerebral desaturation during cardiac surgery was significantly associated with early postoperative neurocognitive dysfunction [80]. However, the neurocognitive test applied (Mini Mental State Examination) is not designed to interrogate the area of the brain that oximeter sensors evaluate, the frontal lobe. CO was primarily designed to interrogate the anterior cerebral circulation, thereby ignoring important neurological structures that impact postoperative neurocognitive performance [81]. Ultimately, it is important to emphasize that besides the known technical difficulties of cerebral oximetry application, the lack of large prospective studies supporting validation or demonstrating long-term benefit in neurologic outcome may preclude its routine use of cerebral oximetry in cardiac surgical patients.
Future directions
Prevention of adverse outcomes has been shown to be more effective than any interventional protocol. Cerebral monitoring has been postulated to identify those clinical conditions that may put a patient’s brain at risk [82, 83]. A tailored, multimodal approach to cerebral monitoring incorporating NIRS technology may permit individualized manipulation of these conditions, consequently improving neurological outcomes [84]. In the specific context of cardiac surgery, research efforts are being coordinated not only to improve this technology but also to expand its applicability and maximize its potential benefit [85]. Badner et al. describe the use of NIRS technology in two case reports to detect spinal cord ischemia by direct application of sensors to the back [86].
Despite limitations and a tremendous amount of work ahead, CO has been investigated to have an additional role in the preoperative risk stratification of cardiac surgical patients (scoring systems), in describing oxygen desaturation in patients undergoing deep hypothermic circulatory arrest or off-pump coronary artery bypass surgery, as well as in perhaps providing a tool to interrogate somatic oxygen saturation [87, 88].
Do redakce došlo dne 8. 6. 2012.
Do tisku přijato dne 30. 6. 2012.
Corresponding Author:
George Silvay, MD, PhD
The Mount Sinai School of Medicine,
One Gustave L. Levy Place, Box 1010
New York, NY 10029-6574, USA
Email: george.silvay@msnyuhealth.org
javier.castillo@mountsinai.org
Zdroje
1. Filsoufi, F., Rahmanian, P. B., Castillo, J. G., Bronster, D., Adams, D. H. Incidence, topography, predictors and long- -term survival after stroke in patients undergoing coronary artery bypass grafting. Ann. Thorac. Surg., 2008, 85, p. 862–870.
2. Newman, M. F., Kirchner, J. L., Phillips-Bute, B., Gaver, V., Grocott, H., Jones, R. H., Mark, D. B., Reves, J. G., Blumenthal, J. A. Longitudinal assessment of neurocognitive function after coronary-artery bypass surgery. N. Engl. J. Med., 2001, 344, p. 395–402.
3. Steinbrook, R. Public report cards – cardiac surgery and beyond. N. Engl. J. Med., 2006, 355, p. 1847–1849.
4. de Vries, J. W., Visser, G. H., Bakker, P. F. Neuromonitoring in defibrillation threshold testing. A comparison between eeg, near-infrared spectroscopy and jugular bulb oximetry. J. Clin. Monit., 1997, 13, p. 303–307.
5. Schwarz, G., Litscher, G. Transcranial cerebral oximetry, transcranial doppler sonography, and heart rate variability: Useful neuromonitoring tools in anaesthesia and intensive care? Eur. J. Anaesthesiol., 2002, 19, p. 543–549.
6. Murkin, J. M., Arango, M. Near-infrared spectroscopy as an index of brain and tissue oxygenation. Br. J. Anaesth., 2009, 103 Suppl 1:i3–13.
7. McCormick, P. W., Stewart, M., Goetting, M. G., Dujovny, M., Lewis, G., Ausman, J. I. Noninvasive cerebral optical spectroscopy for monitoring cerebral oxygen delivery and hemodynamics. Crit. Care Med., 1991, 19, p. 89–97.
8. Benaron, D. A., Parachikov, I. H., Cheong, W. F., Friedland, S., Rubinsky, B. E., Otten, D. M., Liu, F. W., Levinson, C. J., Murphy, A. L., Price, J. W., Talmi, Y., Weersing, J. P., Duckworth, J. L., Horchner, U. B., Kermit, E. L. Design of a visible-light spectroscopy clinical tissue oximeter. J. Biomed. Opt., 2005, 10, 44–45.
9. Germon, T. J., Evans, P. D., Barnett, N. J., Wall, P., Manara, A. R., Nelson, R. J. Cerebral near infrared spectroscopy: Emitter-detector separation must be increased. Br. J. Anaesth., 1999, 82, p. 831–837.
10. Banaji, M., Mallet, A., Elwell, C. E., Nicholls, P., Cooper, C. E. A model of brain circulation and metabolism: Nirs signal changes during physiological challenges. PLoS Comput. Biol., 2008, 4:e1000212.
11. Trivedi, N. S., Ghouri, A. F., Lai, E., Shah, N. K., Barker, S. J. Pulse oximeter performance during desaturation and resaturation: A comparison of seven models. J. Clin. Anesth., 1997, 9, p. 184–188.
12. Pattinson, K. T., Imray, C. H., Wright, A. D. What does cerebral oximetry measure? Br. J. Anaesth., 2005, 94, 863, author reply p. 863–864.
13. Gratton, E., Toronov, V., Wolf, U., Wolf, M., Webb, A. Measurement of brain activity by near-infrared light. J. Biomed. Opt., 2005, 10, 11008.
14. Wolf, M., Wolf, U., Toronov, V., Michalos, A., Paunescu, L. A., Choi, J. H., Gratton, E. Different time evolution of oxyhemoglobin and deoxyhemoglobin concentration changes in the visual and motor cortices during functional stimulation: A near-infrared spectroscopy study. Neuroimage, 2002, 16, p. 704–712.
15. Nelson, L. A., McCann, J. C., Loepke, A. W., Wu, J., Ben Dor, B., Kurth, C. D. Development and validation of a multiwavelength spatial domain near-infrared oximeter to detect cerebral hypoxia-ischemia. J. Biomed. Opt., 2006, 11, 064022.
16. Tisdall, M. M., Tachtsidis, I., Leung, T. S., Elwell, C. E., Smith, M. Near-infrared spectroscopic quantification of changes in the concentration of oxidized cytochrome c oxidase in the healthy human brain during hypoxemia. J. Biomed. Opt., 2007, 12:024002.
17. Al-Rawi, P. G., Smielewski, P., Kirkpatrick, P. J. Evaluation of a near-infrared spectrometer (niro 300) for the detection of intracranial oxygenation changes in the adult head. Stroke, 2001, 32, p. 2492–2500.
18. Wahr, J. A., Tremper, K. K., Samra, S., Delpy, D. T. Near- -infrared spectroscopy: Theory and applications. J. Cardiothorac. Vasc. Anesth., 1996, 10, p. 406–418.
19. Yoshitani, K., Kawaguchi, M., Miura, N., Okuno, T., Kanoda, T., Ohnishi, Y., Kuro, M. Effects of hemoglobin concentration, skull thickness, and the area of the cerebrospinal fluid layer on near-infrared spectroscopy measurements. Anesthesiology, 2007, 106, p. 458–462.
20. Maniewski, R., Liebert, A., Kacprzak, M. Time resolved optical spectroscopy system for cerebral oxygenation imaging. Conf. Proc. IEEE Eng. Med. Biol. Soc., 2005, 1, p. 201–204.
21. Moller, J. T., Pedersen, T., Rasmussen, L. S., Jensen, P. F., Pedersen, B. D., Ravlo, O., Rasmussen, N. H., Espersen, K., Johannessen, N. W., Cooper, J. B. et al. Randomized evaluation of pulse oximetry in 20,802 patients: I. Design, demography, pulse oximetry failure rate, and overall complication rate. Anesthesiology, 1993;78:436-444
22. Hoffman, G. M. Neurologic monitoring on cardiopulmonary bypass: What are we obligated to do? Ann. Thorac. Surg., 2006, 81, S2373–2380.
23. Macmillan, C. S., Andrews, P. J. Cerebrovenous oxygen saturation monitoring: Practical considerations and clinical relevance. Intensive Care Med., 2000, 26, p. 1028–1036.
24. Pedersen, T. Does perioperative pulse oximetry improve outcome? Seeking the best available evidence to answer the clinical question. Best Pract. Res. Clin. Anaesthesiol., 2005, 19, p. 111-123–
25. Shaaban Ali, M., Harmer, M., Latto, I. Jugular bulb oximetry during cardiac surgery. Anaesthesia, 2001, 56, p. 24–37.
26. Yoshitani, K., Kawaguchi, M., Iwata, M., Sasaoka, N., Inoue, S., Kurumatani, N., Furuya, H. Comparison of changes in jugular venous bulb oxygen saturation and cerebral oxygen saturation during variations of haemoglobin concentration under propofol and sevoflurane anaesthesia. Br. J. Anaesth., 2005, 94, p. 341–346.
27. Orihashi, K., Sueda, T., Okada, K., Imai, K. Malposition of selective cerebral perfusion catheter is not a rare event. Eur. J. Cardiothorac. Surg., 2005, 27, p. 644–648.
28. Janelle, G. M., Mnookin, S., Gravenstein, N., Martin, T. D., Urdaneta, F. Unilateral cerebral oxygen desaturation during emergent repair of a debakey type 1 aortic dissection: Potential aversion of a major catastrophe. Anesthesiology, 2002, 96, p. 1263–1265.
29. Han, S. H., Kim, C. S., Lim, C., Kim, W. H. Obstruction of the superior vena cava cannula detected by desaturation of the cerebral oximeter. J. Cardiothorac. Vasc. Anesth., 2005, 19, p. 420–421.
30. Sakamoto, T., Duebener, L. F., Laussen, P. C., Jonas, R. A. Cerebral ischemia caused by obstructed superior vena cava cannula is detected by near-infrared spectroscopy. J. Cardiothorac. Vasc. Anesth., 2004, 18, p. 293–303.
31. Germon, T. J., Kane, N. M., Manara, A. R., Nelson, R. J. Near-infrared spectroscopy in adults: Effects of extracranial ischaemia and intracranial hypoxia on estimation of cerebral oxygenation. Br. J. Anaesth., 1994, 73, p. 503–506.
32. Papadimos, T. J., Marco, A. P. Cerebral oximetry and an unanticipated circulatory arrest. Anaesthesia, 2004, 59, p. 309–310.
33. Prabhune, A., Sehic, A., Spence, P. A., Church, T., Edmonds, H. L., Jr. Cerebral oximetry provides early warning of oxygen delivery failure during cardiopulmonary bypass. J. Cardiothorac. Vasc. Anesth., 2002, 16, p. 204–206.
34. Yeh, T., Jr., Austin, E. H., Sehic, A., Edmonds, H. L., Jr. Rapid recognition and treatment of cerebral air embolism: The role of neuromonitoring. J. Thorac. Cardiovasc. Surg., 2003, 126, p. 589–591.
35. Fischer, G. W., Stone, M. E. Cerebral air embolism recognized by cerebral oximetry. Semin. Cardiothorac. Vasc. Anesth., 2009, 13, p. 56–59.
36. Moritz, S., Rochon, J., Volkel, S., Hilker, M., Hobbhahn, J., Graf, B. M., Arlt, M. Determinants of cerebral oximetry in patients undergoing off-pump coronary artery bypass grafting: An observational study. Eur. J. Anaesthesiol., 2010, 27, p. 542–549.
37. Talpahewa, S. P., Lovell, A. T., Angelini, G. D., Ascione, R. Effect of cardiopulmonary bypass on cortical cerebral oxygenation during coronary artery bypass grafting. Eur. J. Cardiothorac. Surg., 2004, 26, p. 676–681.
38. Alie, R. F., Hymes, W., Kooperman, S. Intra-aortic balloon counterpulsation in an off-pump procedure shows improved cerebral perfusion by near-infrared spectroscopy. Journal of cardiothoracic and vascular anesthesia, 2010, 24, p. 300–302.
39. Heringlake, M., Garbers, C., Kabler, J. H., Anderson, I., Heinze, H., Schon, J., Berger, K. U., Dibbelt, L., Sievers, H. H., Hanke, T. Preoperative cerebral oxygen saturation and clinical outcomes in cardiac surgery. Anesthesiology, 2011, 114, p. 58–69.
40. MacLeod, D. Simultaneous comparison of fore-sight and invos cerebral oximeters to jugular bulb and arterial co-oximetry measurements in healthy volunteers. 31st Annual Meeeting of the Society of Cardiovascular Anesthesiologists, 2009.
41. Tobias, J. D. Cerebral oximetry monitoring with near infrared spectroscopy detects alterations in oxygenation before pulse oximetry. J. Intensive Care Med., 2008, 23, p. 384–388.
42. Hock, C., Muller-Spahn, F., Schuh-Hofer, S., Hofmann, M., Dirnagl, U., Villringer, A. Age dependency of changes in cerebral hemoglobin oxygenation during brain activation: A near-infrared spectroscopy study. J. Cereb. Blood Flow Metab., 1995, 15, p. 1103–1108.
43. Kishi, K., Kawaguchi, M., Yoshitani, K., Nagahata, T., Furuya, H. Influence of patient variables and sensor location on regional cerebral oxygen saturation measured by invos 4100 near-infrared spectrophotometers. J. Neurosurg. Anesthesiol., 2003, 15, p. 302–306.
44. Lassnigg, A., Hiesmayr, M., Keznickl, P., Mullner, T., Ehrlich, M., Grubhofer, G. Cerebral oxygenation during cardiopulmonary bypass measured by near-infrared spectroscopy: Effects of hemodilution, temperature, and flow. J. Cardiothorac. Vasc. Anesth., 1999, 13, p. 544–548.
45. Marimon, G. A., Dockery, W. K., Sheridan, M. J., Agarwal, S. Near-infrared spectroscopy cerebral and somatic (renal) oxygen saturation correlation to continuous venous oxygen saturation via intravenous oximetry catheter. J. Crit. Care, 2012, 27, 314, e313–318.
46. Baraka, A., Naufal, M., El-Khatib, M. Correlation between cerebral and mixed venous oxygen saturation during moderate versus tepid hypothermic hemodiluted cardiopulmonary bypass. J. Cardiothorac. Vasc. Anesth., 2006, 20, p. 819–825.
47. O’Dwyer, C., Prough, D. S., Johnston, W. E. Determinants of cerebral perfusion during cardiopulmonary bypass. J. Cardiothorac. Vasc. Anesth., 1996, 10, p. 54–64; quiz 65.
48. Davie, S. N., Grocott, H. P. Impact of extracranial contamination on regional cerebral oxygen saturation: A comparison of three cerebral oximetry technologies. Anesthesiology, 2012, 116, p. 834–840.
49. Vohra, H. A., Modi, A., Ohri, S. K. Does use of intra- -operative cerebral regional oxygen saturation monitoring during cardiac surgery lead to improved clinical outcomes? Interact. Cardiovasc. Thorac. Surg., 2009, 9, p. 318–322.
50. Pringle, J., Roberts, C., Kohl, M., Lekeux, P. Near infrared spectroscopy in large animals: Optical pathlength and influence of hair covering and epidermal pigmentation. Vet. J., 1999, 158, p. 48–52.
51. Thavasothy, M., Broadhead, M., Elwell, C., Peters, M., Smith, M. A comparison of cerebral oxygenation as measured by the niro 300 and the invos 5100 near-infrared spectrophotometers. Anaesthesia, 2002, 57, p. 999–1006.
52. Toraman, F. Comparison of two different devices for measurement of regional cerebral oxygen saturation. 25th Annual Meeting of the European Association of Cardiothoracic Anesthesiologists, 2010.
53. Yoshitani, K., Kawaguchi, M., Tatsumi, K., Kitaguchi, K., Furuya, H. A comparison of the invos 4100 and the niro 300 near-infrared spectrophotometers. Anesth. Analg., 2002, 94, p. 586–590; table of contents.
54. Misra, M., Stark, J., Dujovny, M., Widman, R., Ausman, J. I. Transcranial cerebral oximetry in random normal subjects. Neurol. Res., 1998, 20, p. 137–141.
55. Edmonds, H. L., Jr., Ganzel, B. L., Austin, E. H. Cerebral oximetry for cardiac and vascular surgery. Semin. Cardiothorac. Vasc. Anesth., 2004, 8, p. 147–166.
56. Taillefer, M. C., Denault, A. Y. Cerebral near-infrared spectroscopy in adult heart surgery: Systematic review of its clinical efficacy. Can. J. Anaesth., 2005, 52, p. 79–87.
57. Murkin, J. M., Adams, S. J., Novick, R. J., Quantz, M., Bainbridge, D., Iglesias, I., Cleland, A., Schaefer, B., Irwin, B., Fox, S. Monitoring brain oxygen saturation during coronary bypass surgery: A randomized, prospective study. Anesth. Analg., 2007, 104, p. 51–58.
58. Sakamoto, T., Kurosawa, H., Shin’oka, T., Aoki, M., Isomatsu, Y. The influence of ph strategy on cerebral and collateral circulation during hypothermic cardiopulmonary bypass in cyanotic patients with heart disease: Results of a randomized trial and real-time monitoring. J. Thorac. Cardiovasc. Surg., 2004, 127, p. 12–19.
59. Murkin, J. M. Cerebral oximetry: Monitoring the brain as the index organ. Anesthesiology, 2011, 114, p. 12–13.
60. Slater, J. P., Guarino, T., Stack, J., Vinod, K., Bustami, R. T., Brown, J. M., Rodriguez, A. L., Magovern, C. J., Zaubler, T., Freundlich, K., Parr, G. V. Cerebral oxygen desaturation predicts cognitive decline and longer hospital stay after cardiac surgery. Ann. Thorac. Surg., 2009, 87, p. 36–44; discussion p. 44–35.
61. Fischer, G. W., Lin, H. M., Krol, M., Galati, M. F., Di Luozzo, G., Griepp, R. B., Reich, D. L. Noninvasive cerebral oxygenation may predict outcome in patients undergoing aortic arch surgery. The Journal of thoracic and cardiovascular surgery, 2011, 141, p. 815–821.
62. Fischer, G. W., Benni, P. B., Lin, H. M., Satyapriya, A., Afonso, A., Di Luozzo, G., Griepp, R. B., Reich, D. L. Mathematical model for describing cerebral oxygen desaturation in patients undergoing deep hypothermic circulatory arrest. British Journal of Anaesthesia, 2010, 104, p. 59–66.
63. Rifai, L., Winters, J., Friedman, E., Silver, M. A. Initial description of cerebral oximetry measurement in heart failure patients. Congest. Heart Fail., 2012, 18, p. 85–90.
64. Anastasiadis, K., Argiriadou, H., Antonitsis, P., Chalvatzoulis, O., Papakonstantinou, C. Cerebral oximetry-guided antegrade cerebral perfusion in aortic arch surgery. Journal of Cardiothoracic and Vascular Anesthesia, 2011, 25, p. 591–592.
65. Santo, K. C., Barrios, A., Dandekar, U., Riley, P., Guest, P., Bonser, R. S. Near-infrared spectroscopy: An important monitoring tool during hybrid aortic arch replacement. Anesth. Analg., 2008, 107, p. 793–796.
66. Steiner, L. A., Pfister, D., Strebel, S. P., Radolovich, D., Smielewski, P., Czosnyka, M. Near-infrared spectroscopy can monitor dynamic cerebral autoregulation in adults. Neurocrit. Care, 2009, 10, p. 122–128.
67. Davies, L. K., Janelle, G. M. Con: All cardiac surgical patients should not have intraoperative cerebral oxygenation monitoring. J. Cardiothorac. Vasc. Anesth., 2006, 20, p. 450–455.
68. Edmonds, H. L., Jr. All cardiac surgical patients should have intraoperative cerebral oxygenation monitoring. J. Cardiothorac. Vasc. Anesth., 2006, 20, p. 445–449.
69. Hoffman, G. M. Near-infrared spectroscopy should be used for all cardiopulmonary bypass. J. Cardiothorac. Vasc. Anesth., 2006, 20, p. 606–612.
70. Grocott, H. P., Homi, H. M., Puskas, F. Cognitive dysfunction after cardiac surgery: Revisiting etiology. Semin. Cardiothorac. Vasc. Anesth., 2005, 9, p. 123–129.
71. Leggat, C. S., Fischer, G. W. Early detection of an acute cerebral event during cardiopulmonary bypass using a bispectral index monitor. Semin. Cardiothorac. Vasc. Anesth., 2008, 12, p. 80–82.
72. Cohn, S. M., Nathens, A. B., Moore, F. A., Rhee, P., Puyana, J. C., Moore, E. E., Beilman, G. J. Tissue oxygen saturation predicts the development of organ dysfunction during traumatic shock resuscitation. J. Trauma, 2007, 62, p. 44–54; discussion 54–45.
73. Ferrari, M., Mottola, L., Quaresima, V. Principles, techniques, and limitations of near infrared spectroscopy. Can. J. Appl. Physiol., 2004, 29, p. 463–487.
74. Dullenkopf, A., Baulig, W., Weiss, M., Schmid, E. R. Cerebral near-infrared spectroscopy in adult patients after cardiac surgery is not useful for monitoring absolute values but may reflect trends in venous oxygenation under clinical conditions. J. Cardiothorac. Vasc. Anesth., 2007, 21, p. 535–539.
75. Baulig, W. Cerebral oxygen saturation measured by near-infrared-spectroscopy does not reliably detect the cerebral status. 25th Annual Meeting of the European Association of Cardiothoracic Anesthesiologists, 2010.
76. Schwarz, G., Litscher, G., Kleinert, R., Jobstmann, R. Cerebral oximetry in dead subjects. J. Neurosurg. Anesthesiol., 1996, 8, p. 189–193.
77. Maeda, H., Fukita, K., Oritani, S., Ishida, K., Zhu, B. L. Evaluation of post-mortem oxymetry with reference to the causes of death. Forensic. Sci. Int., 1997, 87, p. 201–210.
78. Al-Rawi, P. G., Kirkpatrick, P. J. Tissue oxygen index: Thresholds for cerebral ischemia using near-infrared spectroscopy. Stroke, 2006, 37, p. 2720–2725.
79. Kirkpatrick, P. J., Lam, J., Al-Rawi, P., Smielewski, P., Czosnyka, M. Defining thresholds for critical ischemia by using near-infrared spectroscopy in the adult brain. J. Neurosurg., 1998, 89, p. 389–394.
80. Yao, F. S., Tseng, C. C., Ho, C. Y., Levin, S. K., Illner, P. Cerebral oxygen desaturation is associated with early postoperative neuropsychological dysfunction in patients undergoing cardiac surgery. J. Cardiothorac. Vasc. Anesth., 2004, 18, p. 552–558.
81. Gottesman, R. F., Sherman, P. M., Grega, M. A., Yousem, D. M., Borowicz, L. M., Jr., Selnes, O. A., Baumgartner, W. A., McKhann, G. M. Watershed strokes after cardiac surgery: Diagnosis, etiology, and outcome. Stroke, 2006, 37, p. 2306–2311.
82. Maas, A. I., Citerio, G. Noninvasive monitoring of cerebral oxygenation in traumatic brain injury: A mix of doubts and hope. Intensive Care Med., 2010.
83. Paarmann, H., Heringlake, M., Sier, H., Schoen, J. The association of non-invasive cerebral and mixed venous oxygen saturation during cardiopulmonary resuscitation. Interact. Cardiovasc. Thorac. Surg., 2010.
84. Heringlake, M., Heinze, H., Misfeld, M., Klaus, S., Bechtel, M. Goal-directed hemodynamic optimization in high-risk cardiac surgery patients: A tale from the past or a future obligation? Minerva Anestesiol., 2008, 74, p. 251–258.
85. Hargroves, D. R., Tallis, R. C., Pomeroy, V. M., Bhalla, A. Near-infrared spectroscopy in stroke: From research to clinical practice. Stroke, 2004, 35, 2430; author reply p. 2430–2431.
86. Badner, N. H., Nicolaou, G., Clarke, C. F., Forbes, T. L. Use of spinal near-infrared spectroscopy for monitoring spinal cord perfusion during endovascular thoracic aortic repairs. Journal of cardiothoracic and vascular anesthesia, 2011, 25, p. 316–319.
87. Joshi, B., Brady, K., Lee, J., Easley, B., Panigrahi, R., Smielewski, P., Czosnyka, M., Hogue, C. W., Jr. Impaired autoregulation of cerebral blood flow during rewarming from hypothermic cardiopulmonary bypass and its potential association with stroke. Anesth. Analg., 2010, 110, p. 321–328.
88. Lee, J. K., Kibler, K. K., Benni, P. B., Easley, R. B., Czosnyka, M., Smielewski, P., Koehler, R. C., Shaffner, D. H., Brady, K. M. Cerebrovascular reactivity measured by near-infrared spectroscopy. Stroke, 2009, 40, p. 1820–1826.
Štítky
Anestéziológia a resuscitácia Intenzívna medicínaČlánok vyšiel v časopise
Anesteziologie a intenzivní medicína
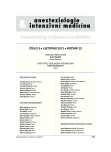
2012 Číslo 5
- MUDr. Lenka Klimešová: Multiodborová vizita je kľúč k efektívnejšej perioperačnej liečbe chronickej bolesti
- Realita liečby bolesti v paliatívnej starostlivosti v Nemecku
- e-Konzilium.cz — Masivní plicní embolie při tromboembolické nemoci
- Kvalita výživy na JIS a následná kvalita života spolu úzko súvisia
- DESATORO PRE PRAX: Aktuálne odporúčanie ESPEN pre nutričný manažment u pacientov s COVID-19
Najčítanejšie v tomto čísle
- Perforated peptic ulcer – pre-operative risks, postoperative morbidity and mortality, prophylaxis in intensive care and guidelines
- Doporučení pro ochranu očí v průběhu celkové anestezie
- Sugammadex from the perspective of evidence-based medicine
- Zemřel MUDr. Vladimír Lemon