Tumor Microenvironment – Possibilities of the Research Under In Vitro Conditions
Authors:
P. Chlapek 1; S. Chovanová 1,2; V. Sláviková 1; R. Veselska 1,3
Authors‘ workplace:
Laboratoř nádorové biologie, Ústav experimentální biologie, Přírodovědecká fakulta MU, Brno
1; Regionální centrum aplikované molekulární onkologie, Masarykův onkologický ústav, Brno
2; Klinika dětské onkologie LF MU a FN Brno
3
Published in:
Klin Onkol 2014; 27(Supplementum): 48-52
Overview
Tumorigenesis is always accompanied by alterations of the microenvironment in the respective tissue. The tumor microenvironment represents a heterogeneous complex, in which cell proliferation, differentiation, necrosis or apoptosis are regulated by various extracellular stimuli, and it can also lead to development of an aggressive phenotype of tumor cells. Influence of tumor microenvironment is also often connected with resistance to frequently used therapeutic procedures. Specifics of the tumor microenvironment are closely associated with the structural and functional abnormalities of tumor microvessels and altered cellular metabolism. Moreover, changes such as increase in glycolysis, elevated glucose uptake, production of lactate and CO2, and presence of hypoxic regions and regions with acidic pH are typical features of tumor tissues. At present, there is a lot of methods for in vitro simulation and investigation of some of these specific conditions, and a number of new methods are being developed. A detailed understanding of the specifics of the tumor microenvironment should increasingly improve the development of new treatment possibilities of human cancers.
Key words:
tumor microenvironment – hypoxia – acidic pH – glucose deprivation – in vitro conditions
This study was supported by project CEB:
OP VK CZ.1.07/2.3.00/20.0183), by project MUNI/C/0944/2013 and by the European Regional Development Fund and the State Budget of the Czech Republic (RECAMO; CZ.1.05./2.1.00/03.0101).
The authors declare they have no potential conflicts of interest concerning drugs, products, or services used in the study.
The Editorial Board declares that the manuscript met the ICMJE “uniform requirements” for biomedical papers.
Submitted:
15. 1. 2014
Accepted:
5. 5. 2014
Sources
1. Hanahan D, Weinberg RA. Hallmarks of cancer: the next generation. Cell 2011; 144(5): 646– 674. doi: 10.1016/ j.cell.2011.02.013.
2. Nowell PC. Tumor progression: a brief historical perspective. Semin Cancer Biol 2002; 12(4): 261– 266.
3. Weinberg RA. The biology of cancer. New York: Garland Science 2007.
4. Gillies RJ, Morse DL. In vivo magnetic resonance spectroscopy in cancer. Annu Rev Biomed Eng 2005; 7: 287– 326.
5. Dang CV. Links between metabolism and cancer. Genes Dev 2012; 26(9): 877– 890. doi: 10.1101/ gad.189365.112.
6. Heiden MG, Cantley LC, Thompson CB. Understanding the warburg effect: the metabolic requirements of cell proliferation. Science 2009; 324(5930): 1029– 1033.
7. Kroemer G, Pouyssegur J. Tumor cell metabolism: cancer‘s Achilles‘ heel. Cancer Cell 2008; 13(6): 472– 482.
8. Hamanaka RB and Chandel NS. Targeting glucose metabolism for cancer therapy. J Exp Med 2012; 209(2): 211– 215.
9. Lunt SY, Vander Heiden MG. Aerobic glycolysis: meeting the metabolic requirements of cell proliferation. Annu Rev Cell Dev Biol 2011; 27: 441– 464. doi: 10.1146/ annurev‑ cellbio‑ 092910-154237.
10. Meijer TW, Kaanders JH, Span PN et al. Targeting hypoxia, HIF‑ 1, and tumor glucose metabolism to improve radiotherapy efficacy. Clin Cancer Res 2012; 18(20): 5585– 5594. doi: 10.1158/ 1078- 0432.CCR‑ 12- 0858.
11. Hirschhaeuser F, Sattler UG, Mueller‑ Klieser W. Lactate: a metabolic key player in cancer. Cancer Res 2011; 71(22): 6921– 6925. doi: 10.1158/ 0008- 5472.CAN‑ 11- 1457.
12. Cairns R, Papandreou I, Denko N. Overcoming physiologic barriers to cancer treatment by molecularly targeting the tumor microenvironment. Mol Cancer Res 2006; 4(2): 61– 70.
13. Helmlinger G, Sckell A, Dellian M et al. Acid production in glycolysis‑ impaired tumors provides new insights into tumor metabolism. Clin Cancer Res 2002; 8(4): 1284– 1291.
14. Volk T, Jähde E, Fortmeyer HP et al. pH in human tumour xenografts: effect of intravenous administration of glucose. Br J Cancer 1993; 68(3): 492– 500.
15. Zhang X, Lin Y, Gillies RJ. Tumor pH and its measurement. J Nucl Med 2010; 51(8): 1167– 1170. doi: 10.2967/ jnumed.109.068981.
16. Höckel M, Schlenger K, Knoop C et al. Oxygenation of carcinomas of the uterine cervix: evaluation by computerized O2 tension measurements. Cancer Res 1991; 51(22): 6098– 6102.
17. Rademakers SE, Span PN, Kaanders JH et al. Molecular aspects of tumour hypoxia. Mol Oncol 2008; 2(1): 41– 53. doi: 10.1016/ j.molonc.2008.03.006.
18. Zhu W, Dai M, Xu Y et al. Novel nitroheterocyclic hypoxic markers for solid tumor: synthesis and biological evaluation. Bioorg Med Chem 2008; 16(6): 3255– 3260.
19. Plathow C, Weber WA. Tumor cell metabolism imaging. J Nucl Med 2008; 49 (Suppl 2): 43S–63S. doi: 10.2967/ jnumed.107.045930.
20. Walker‑ Samuel S, Ramasawmy R, Torrealdea F et al.In vivo imaging of glucose uptake and metabolism in tumors. Nat Med 2013; 19(8): 1067– 1072. doi: 10.1038/ nm.3252.
21. Ivanovic Z. Hypoxia or in situ normoxia: the stem cell paradigm. J Cell Physiol 2009; 219(2): 271– 275. doi: 10.1002/ jcp.21690.
22. Vaupel P, Mayer A, Höckel M. Tumor hypoxia and malignant progression. Methods Enzymol 2004; 381: 335– 354.
23. Wykoff CC, Beasley N, Watson PH et al. Expression of the hypoxia‑ inducible and tumor‑associated carbonic anhydrases in ductal carcinoma in situ of the breast. Am J Pathol 2001; 158(3): 1011– 1019.
24. Gatenby RA, Gillies RJ. Why do cancers have high aerobic glycolysis? Nat Rev Cancer 2004; 4(11): 891– 899.
25. Annibaldi A, Widmann C. Glucose metabolism in cancer cells. Curr Opin Clin Nutr Metab Care 2010; 13(4): 466– 470. doi: 10.1097/ MCO.0b013e32833a5577.
26. Furuta E, Pai SK, Zhan R et al. Fatty acid synthase gene is up‑ regulated by hypoxia via activation of Akt and sterol regulatory element binding protein‑1. Cancer Res 2008; 68(4): 1003– 1011. doi: 10.1158/ 0008- 5472.CAN‑ 07- 2489.
27. Hochachka PW, Buck LT, Doll CJ et al. Unifying theory of hypoxia tolerance: molecular/ metabolic defense and rescue mechanisms for surviving oxygen lack. Proc Natl Acad Sci USA 1996; 93(18): 9493– 9498.
28. Kuhajda FP, Jenner K, Wood FD et al. Fatty acid synthesis: a potential selective target for antineoplastic therapy. Proc Natl Acad Sci USA 1994; 91(14): 6379– 6383.
29. Glazer PM, Hegan DC, Lu Y et al. Hypoxia and DNA Repair. Yale J Biol Med 2013; 86(4): 443– 451.
30. Vaupel P, Höckel M, Mayer A. Detection and characterization of tumor hypoxia using pO2 histography. Antioxid Redox Signal 2007; 9(8): 1221– 1235.
31. Lemaire L, Howe FA, Rodrigues LM et al. Assessment of induced rat mammary tumour response to chemotherapy using the apparent diffusion coefficient of tissue water as determined by diffusion‑ weighted (1)H‑ NMR spectroscopy in vivo. MAGMA 1999; 8(1): 20– 26.
32. Gray LH, Conger AD, Ebert M et al. The concentration of oxygen dissolved in tissues at the time of irradiation as a factor in radiotherapy. Br J Radiol 1953; 26(312): 638– 648.
33. Teicher BA, Holden SA, Al‑ Achi A et al. Classification of antineoplastic treatments by their differential toxicity toward putative oxygenated and hypoxic tumor subpopulations in vivo in the FSaIIC murine fibrosarcoma. Cancer Res 1990; 50(11): 3339– 3344.
34. Saikumar P, Dong Z, Patel Y et al. Role of hypoxia‑induced Bax translocation and cytochrome c release in reoxygenation injury. Oncogene 1998; 17(26): 3401– 3415.
35. Dong Z, Venkatachalam MA, Wang J et al. Up‑ regulation of apoptosis inhibitory protein IAP‑ 2 by hypoxia. Hif‑ 1- independent mechanisms. J Biol Chem 2001; 276(22): 18702– 18709.
36. Cipolleschi MG, D‘Ippolito G, Bernabei PA et al. Severe hypoxia enhances the formation of erythroid bursts from human cord blood cells and the maintenance of BFU‑ E in vitro. Exp Hematol 1997; 25(11): 1187– 1194.
37. Cipolleschi MG, Rovida E, Dello Sbarba P. The culture‑repopulating ability assays and incubation in low oxygen: a simple way to test drugs on leukaemia stem or progenitor cells. Curr Pharm Des 2013; 19(30): 5374– 5383.
38. Eliasson P, Jönsson JI. The hematopoietic stem cell niche: low in oxygen but a nice place to be. J Cell Physiol 2010; 222(1): 17– 22. doi: 10.1002/ jcp.21908.
39. Cipolleschi MG, Marzi I, Santini R et al. Hypoxia‑resistant profile implies vulnerability of cancer stem cells to physiological agents, which suggests new therapeutic targets. Cell Cycle 2013; 13(2): 268– 278. doi: 10.4161/ cc.27031.
40. Anderson KM, Guinan P, Rubenstein M. Normoxic or hypoxic CD44/ CD41 a(2) B(1) integrin‑positive prostate PC3 cell side fractions and cancer stem cells. Med Oncol 2014; 31(1): 779. doi: 10.1007/ s12032- 013- 0779- 1.
41. Mahoney BP, Raghunand N, Baggett B et al. Tumor acidity, ion trapping and chemotherapeutics. I. Acid pH affects the distribution of chemotherapeutic agents in vitro. Biochem Pharmacol 2003; 66(7): 1207– 1218.
42. Danhier F, Feron O, Preat V. To exploit the tumor microenvironment: passive and active tumor targeting of nanocarriers for anti‑cancer drug delivery. J Control Release 2010; 148(2): 135– 146. doi: 10.1016/ j.jconrel.2010.08.027.
43. Justus CR, Dong L, Yang LV. Acidic tumor microenvironment and pH‑ sensing G protein‑coupled receptors. Front Physiol 2013; 4: 354.
44. Cukierman E, Khan DR. The benefits and challenges associated with the use of drug delivery systems in cancer therapy. Biochem Pharmacol 2010; 80(5): 762– 770. doi: 10.1016/ j.bcp.2010.04.020.
45. Svastova E, Hulikova A, Rafajova M et al. Hypoxia activates the capacity of tumor‑associated carbonic anhydrase IX to acidify extracellular pH. FEBS Lett 2004; 577(3): 439– 445.
46. Gillies RJ, Raghunand N, Karczmar GS et al. MRI of the tumor microenvironment. J Magn Reson Imaging 2002; 16(4): 430– 450.
47. Fischer K, Hoffmann P, Voelkl S et al. Inhibitory effect of tumor cell‑ derived lactic acid on human T cells. Blood 2007; 109(9): 3812– 3819.
48. Yuan J, Narayanan L, Rockwell S et al. Diminished DNA repair and elevated mutagenesis in mammalian cells exposed to hypoxia and low pH. Cancer Res 2000; 60(16): 4372– 4376.
49. Morita T, Nagaki T, Fukuda I et al. Clastogenicity of low pH to various cultured mammalian cells. Mutat Res 1992; 268(2): 297– 305.
50. Morita T. Low pH leads to sister‑ chromatid exchanges and chromosomal aberrations, and its clastogenicity is S‑ dependent. Mutat Res 1995; 334(3): 301– 308.
51. Hirayama A, Kami K, Sugimoto M et al. Quantitative metabolome profiling of colon and stomach cancer microenvironment by capillary electrophoresis time‑ of‑ flight mass spectrometry. Cancer Res 2009; 69(11): 4918– 4925. doi: 10.1158/ 0008- 5472.CAN‑ 08- 4806.
52. Urasaki Y, Heath L, Xu CW. Coupling of glucose deprivation with impaired histone H2B monoubiquitination in tumors. PLoS One 2012; 7(5): e36775. doi: 10.1371/ journal.pone.0036775.
53. Seyfried BT, Kiebish M, Marsh J et al. Targeting energy metabolism in brain cancer through calorie restriction and the ketogenic diet. J Cancer Res Ther 2009; 5 (Suppl 1):S7– S15. doi: 10.4103/ 0973- 1482.55134.
54. Skinner R, Trujillo A, Ma X et al. Ketone bodies inhibit the viability of human neuroblastoma cells. J Pediatr Surg 2009; 44(1): 212– 216; discussion 216. doi: 10.1016/ j.jpedsurg.2008.10.042.
55. Jin S, DiPaola RS, Mathew R et al. Metabolic catastrophe as a means to cancer cell death. J Cell Sci 2007; 120(Pt 3):379– 383.
56. Hwang JH, Kim JY, Cha MR et al. Etoposide‑resistant HT‑ 29 human colon carcinoma cells during glucose deprivation are sensitive to piericidin A, a GRP78 down‑ regulator. J Cell Physiol 2008; 215(1): 243– 250.
57. Shen J, Hughes C, Chao C et al. Coinduction of glucose‑regulated proteins and doxorubicin resistance in Chinese hamster cells. Proc Natl Acad Sci USA 1987; 84(10): 3278– 3282.
58. Raffaghello L, Lee C, Safdie FM et al. Starvation‑ dependent differential stress resistance protects normal but not cancer cells against high‑dose chemotherapy. Proc Natl Acad Sci USA 2008; 105(24): 8215– 8220. doi: 10.1073/ pnas.0708100105.
59. Tomida A, Yun J, Tsuruo T. Glucose‑regulated stresses induce resistance to camptothecin in human cancer cells. Int J Cancer 1996; 68(3): 391– 396.
60. Desmaison A, Frongia C, Grenier K et al. Mechanical stress impairs mitosis progression in multi‑cellular tumor spheroids. PLoS One 2013; 8(12): e80447. doi: 10.1371/ journal.pone.0080447.
61. Pampaloni F, Reynaud EG, Stelzer EH. The third dimension bridges the gap between cell culture and live tissue. Nat Rev Mol Cell Biol 2007; 8(10): 839– 845.
62. Horch RE, Boos AM, Quan Y et al. Cancer research by means of tissue engineering – is there a rationale? J Cell Mol Med 2013; 17(10): 1197– 1206. doi: 10.1111/ jcmm.12130.
63. Haycock JW. 3D cell culture: a review of current approaches and techniques. Methods Mol Biol 2011; 695: 1– 15. doi: 10.1007/ 978- 1- 60761- 984- 0_1.
64. Thoma CR, Zimmermann M, Agarkova I et al. 3D cell culture systems modeling tumor growth determinants in cancer target discovery. Adv Drug Deliv Rev 2014; 69– 70C: 29– 41. doi: 10.1016/ j.addr.2014.03.001.
Labels
Paediatric clinical oncology Surgery Clinical oncologyArticle was published in
Clinical Oncology
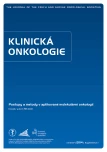
2014 Issue Supplementum
- Metamizole vs. Tramadol in Postoperative Analgesia
- Metamizole at a Glance and in Practice – Effective Non-Opioid Analgesic for All Ages
- Possibilities of Using Metamizole in the Treatment of Acute Primary Headaches
- Current Insights into the Antispasmodic and Analgesic Effects of Metamizole on the Gastrointestinal Tract
- Spasmolytic Effect of Metamizole
Most read in this issue
- Protein Expression and Purification
- Methods for Studying Tumor Cell Migration and Invasiveness
- Next Generation Sequencing – Application in Clinical Practice
- Analysis of Protein Using Mass Spectrometry